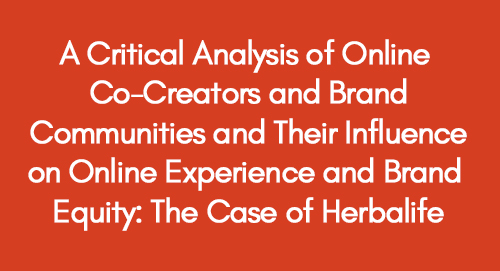
A Critical Analysis of Online Co-Creators and Brand Communities and Their Influence on Online Experience and Brand Equity: The Case of Herbalife
July 12, 2022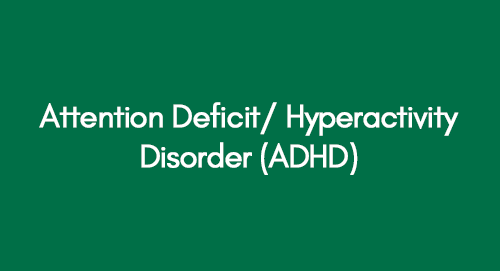
Attention Deficit/ Hyperactivity Disorder (ADHD)
July 12, 2022In the symphony of environmental stewardship, we unravel the Tano River Basin's secrets, employing the artistry of bio-optics modelling and the gaze of remote sensing techniques to meticulously assess the water quality and mineral footprint, orchestrating a ballet of ecological insight.
Outline
- Introduction
- Problem Statement
- Research Aims and Objectives
- Study Area
- Literature Review
- Methodology
- Conclusion
- References
Water, often deemed the elixir of life, is integral to ecosystems and human activities. The Tano River Basin, a vital water resource, sustains communities and biodiversity. Understanding its water quality through bio-optics modelling and remote sensing is crucial for sustainable management. These innovative approaches empower scientists to guide effective conservation efforts amid evolving challenges, ensuring the river's health.
The Tano River Basin stands out as a lifeline in the delicate balance of ecosystems. Bio-optics modelling and remote sensing have become indispensable tools for environmental scientists, providing nuanced insights into water quality. This scientific symphony enables sustainable practices and effective conservation, securing the longevity of this vital natural resource against the backdrop of environmental challenges.
Introduction
Across the globe, water resources are grappling with escalating stressors induced by urbanization, industrial expansion, burgeoning agricultural practices, and the relentless growth of the human population. This heightened strain on water reserves is exacerbated by overexploitation and the intricate interplay of factors linked to global warming. The repercussions of this mounting scarcity extend beyond mere insufficiency, delving into the perilous realm of water contamination within critical catchment areas and the systems designed to deliver clean water. According to Li et al. (2017), the transference of contaminants between different water bodies has become a significant concern. This amalgamation of challenges collectively contributes to the diminishing quality of groundwater, lakes, and rivers, presenting an overarching global dilemma (Behmel et al., 2016; Khan et al., 2013). Reports from esteemed institutions such as the World Health Organization (WHO) and UNICEF Joint Monitoring Programme (JMP) underline the gravity of the situation, revealing that approximately 2 billion people rely on drinking water from sources tainted with faecal matter. Moreover, 785 million people lack access to a fundamental drinking water service (WHO, 2017; UNICEF, 2016). The report further emphasizes that 144 million individuals worldwide collect untreated surface water from lakes, ponds, rivers, and streams (WHO, 2017; UNICEF, 2016).
Mining operations can significantly deteriorate water quality (Northey et al., 2016). Mhlongo et al. (2017) highlight that mining activities pose risks associated with uncontrolled discharges, pit inundation, and dam collapses notorious for causing water pollution. Findings from Gao et al. (2017) underscore the pressing challenges faced by the mining industry, especially in coal mining, concerning water resource management and the prevention of non-compliant water discharges resulting from mining activities. The inadequacy of traditional water storage infrastructure in addressing spill-over from treated water further compounds these challenges. Addressing the risk of unregulated discharges necessitates innovative research, with standardized water quality monitoring being a pivotal step based on systematic observations and measurements of aquatic environments (Behmel et al., 2016). Salem and Amin (2012) point out that water-polluting activities in water catchments compromise biodiversity, food production, human health, and natural ecosystems. Additionally, Khan et al. (2013) report that mining poses a substantial risk to providing clean drinking water to large populations. It is imperative to thoroughly investigate the presence and impact of such activities in water-stressed areas where mining operations occur.
Problem Statement
The mining sector in Ghana plays a pivotal role in its economy, contributing 5% to its GDP and accounting for 37% of total exports, with gold alone making up 90% of mineral exports. The emphasis of the mining and mineral development sector is primarily on gold extraction. However, a significant challenge the industry faces is the utilization of acid mine drainage, leading to the discharge of pollutants into surface water sources and other water bodies. Ghana's Environmental Protection Agency (EPA), Water Resource Commission (WRC), and Municipal Committees oversee and safeguard the Tano River and its Water Basin. In 2010, the WRC issued water use permits for mining and industrial activities in the basin, allowing a total consumption of 70.3 million m3 per year for both surface and groundwater. A critical issue confronting the Tano River and its basin is the surge in illegal mining activities, exacerbating the challenges posed by acid mine drainage—a wastewater effluent generated by mining operations, particularly gold mining.
Despite the magnitude of the issue, there is a scarcity of specialized research employing advanced techniques to comprehensively evaluate the impact of mining on water bodies in Ghana. Previous studies, such as the one by Attiogbe and Nkansah (2017), relied on a mixed-methods model to assess water resources but lacked rigorous techniques to map out the effects of mining in Ghana's waterways. Similarly, studies by Asare-Donkor and Adimado (2016) and Macdonald et al. (2015) focused on measures like mercury levels and physicochemical properties at limited sites. Addressing these gaps, the proposed study aims to employ advanced bio-optical techniques to assess water quality by determining the concentration of Total Suspended Matter (TSM) and chlorophyll-a. Given the dire consequences of mine wastewater effluent, understanding its potential impacts on the Tano River and Water Basin is imperative.
Justification for Research
The communities residing along the Tano River and other areas within the mining catchment zones rely on both groundwater and surface water for drinking and domestic purposes. Consequently, the river's water quality directly influences the well-being of the region's humans, flora, and fauna. Consumption of contaminated water or aquatic organisms can lead to severe health hazards for these communities. Mining activities, including pit digging and runoff, create breeding grounds for disease-carrying insects like mosquitoes, resulting in dengue and malaria. These health hazards persist across regions in Ghana and other parts of Africa, particularly affecting communities near the Tano River and Water Basin. Moreover, mining disrupts natural river flows, contributing to concerns about climate change.
The adverse impacts of mining activities on the Tano River necessitate comprehensive research better to understand the extent of their effects on water bodies. Robust research methodologies are crucial for informing effective policymaking and introducing innovations to address these challenges. Continuous research in this area holds the potential to enhance water quality, consequently improving the quality of life for communities, the local ecosystem, and the environment as a whole.
Research Aims and Objectives
The central objective of this study is to investigate and analyze the patterns of water quality and mineral impact in areas affected by mining and experiencing water stress along the Tano River and Water Basin. To fulfil this overarching goal, the study has delineated specific objectives as follows:
Evaluate the influence of mining operations on the chemical composition of water along the Tano River and Water Basin.
Assess the degree of surface water contamination in the Tano River and Water Basin attributed to mining activities in the designated region, focusing on key physicochemical parameters such as pH, turbidity, total dissolved solids (TDS), and electrical conductivity (EC).
Investigate potential variations in the concentrations of heavy metals within the Tano River, including sulfate, chloride, fluoride, calcium, magnesium, sodium, iron, manganese, and aluminium, exploring the impact of mining activities on these elements.
Utilize multi-sensor images and remote sensing techniques to derive Total Suspended Matter (TSM) concentrations and Chlorophyll-a in the Tano River.
Formulate recommendations for environmental policy adjustments at both local and national levels, drawing upon the insights derived from the outcomes of the proposed study.
The study aims to comprehensively understand the intricate dynamics between mining activities, water quality, and the mineral footprint in the Tano River and Water Basin by addressing these objectives. The ultimate goal is to contribute meaningful recommendations for policy changes impacting the region's environmental sustainability.
Study Area
The research area is situated predominantly between latitudes 6°40' and 7°15' North and longitudes 2°15' and 2°45' West within the Asutifi North District. Geographically, it shares borders with Sunyani Municipal to the North, Tano South District to the North East, Dormaa East District to the North West, Asutifi South District to the West, Asunafo North and South Districts to the South West, and Ahafo Ano South and North Districts (Ashanti Region) to the South East. Spanning a total land surface of 1,500 square kilometres, the district ranks among the smallest in the Brong Ahafo region. The urban areas account for 32% of the district, while the rural areas constitute the remaining 78%. Specifically, the land area of the Asutifi North District is approximately 936.31 square kilometres (Nyantakyi et al., 2013).
The Tano River, a significant watercourse in Ghana, traverses into Cote d’Ivoire (Ivory Coast) and forms a natural international boundary between Ghana and Cote d’Ivoire before eventually reaching the ocean. The river stretches 400 kilometres, passing through the Why Lagoon, Tendo Lagoon, and Aby Lagoon in Cote d’Ivoire before entering the Atlantic Ocean. While the Tano River is navigable up to 95 kilometres from its mouth to Tanoso, further travel is impeded by the Sutre Falls (Asare-Donkor and Adimado, 2016). The primary watershed of Ghana is attributed to the Tano River, along with the parallel Ankobra, draining the western section of a shallow basin situated southwest of the Kwahu Plateau. This region is crucial for various economic activities, notably gold and bauxite mining and timber, palm oil, rubber, and copra production (Owusu-Nimo et al., 2018).
The focus of this study, the Asutifi North District, plays a pivotal role in the Tano River Basin. Inflows from various streams that drain the Ahafo Mines contribute to the Tano River, with the Ahafo Mines being dissected by seasonal streams and rivers into basins like the Amoma and Awonsu basins (Banunle et al., 2018). Between November and April, these basins and their tributaries experience dry conditions. The Tano River was the area's most significant surface water resource throughout this period. It serves as a primary water source for agricultural and domestic activities, with the Ghana Water Company Limited (GWCL) pumping and treating water from the Tano River at Akyerensua. This treated water is then distributed to New Dormaa, Hwidiem, and surrounding areas (Attiogbe and Nkansah, 2017).
Literature Review
In the tapestry of scholarly exploration, the literature review stitches together the threads of existing knowledge, weaving a narrative that guides researchers through the intricate patterns of past inquiries, providing both a compass and a context for the uncharted territories of discovery.
Overview
The population growth and economic advancement surge have placed considerable strain on surface and groundwater systems globally, particularly in arid and semi-arid regions. Ensuring the sustainability of water resources in these areas emerges as a pressing challenge for economic progress. Recognizing the environment as a rightful stakeholder in water resource allocation and acknowledging the pivotal role of ecosystem services in economic assessments underscores the necessity for a paradigm shift in water resources management. This necessitates a fresh approach that comprehensively understands the intricate interactions among the environment, ecosystems, and economic activities. Numerous studies have sought to enhance water resource management, optimising system performance and mitigating environmental pressures by amalgamating natural processes and human activities within a unified analytical framework. The proposed study intends to thoroughly review existing literature, employing a thematic review method to organize available data into coherent themes for a comprehensive understanding of these complex interactions.
Water Resource Management
International Water Resource Management (IWRM) draws inspiration from the principles outlined in the Dublin Statement on Water and Sustainable Development, as declared during the International Conference on Water and the Environment (ICWE) in Dublin in 1992. The foundational principle asserts that "fresh water is a finite and vulnerable resource, essential to sustain life, development, and the environment" (ICWE, 1992). This principle underscores the need for a comprehensive management approach that considers all facets of the hydrological cycle and its interactions with other natural resources and ecosystems and addresses resource demands and threats.
At the core of IWRM lies the hydrological cycle, where rain, a pivotal component, interacts with watersheds through interception by plants, infiltration into the ground, or surface runoff (Chow, 1964). Surface runoff refers to water flowing overland, joining streams, while base flow represents water infiltrating the ground and later contributing to streamflow. The balance between infiltration and surface runoff hinges on factors such as ground porosity, existing soil moisture, rainfall intensity, aridity, vegetation coverage, slope gradient, and the degree of impervious surfaces. This intricate interplay necessitates integrated management strategies bridging both surface and groundwater considerations.
Rivers, dynamic and subject to constant change, undergo alterations due to natural and human-induced factors. Natural changes, gradual and potentially self-regulating, stand in contrast to human-induced alterations that can swiftly upset the equilibrium. Examples like the degradation of the Mesopotamian marshlands in the Tigris and Euphrates river basins highlight the consequences of unsustainable damming and river channelization during the late 1980s (UNEP, 2001). Human activities, such as deforestation and urbanization, further contribute to changes in erosion rates, infiltration, and overland flow, impacting reservoir capacity, inducing downstream flooding, and affecting water quantity and quality due to climate change (IPCC, 2000). The intricate interactions within the hydrologic cycle involve air, soil, vegetation, surface, and groundwater, necessitating the integration of land and water management for a comprehensive understanding and effective management of quantity and quality relationships and upstream and downstream water interests (GWP, 2000).
GWP (2000) emphasizes that successful IWRM implementation relies on management instruments—tools facilitating decision-makers in making coherent choices among alternatives. Among these tools, water resources assessments and the development of knowledge bases are crucial for effective water management, addressing the spatial and temporal occurrence of both surface and groundwater. Irvine et al. (2005) further highlight the need for mathematical models to synthesize complex natural processes and predict responses to natural and anthropogenic changes, particularly in effectively implementing the Water Framework Directive (WFD).
Methodology
In the intricate dance between theory and practice, the methodology takes centre stage as the choreographer of empirical exploration. It is the art and science of orchestrating research, seamlessly blending precision and creativity to unlock the secrets held within the data, guiding the researcher through the labyrinth of investigation with a methodical compass. In this symphony of inquiry, the methodology is both conductor and composer, shaping the harmonious progression of steps that lead to meaningful insights and scholarly contributions.
Water Sampling and Treatment
The data collected for the present study will be acquired from the municipality and Ghana water authority data from the Asutifi North District and the streams that drain the Ahafo Mines. Thirty (30) samples are proposed to be taken with duplicates from access points to the Tano River in the Asutifi North District. It is proposed that samples be obtained at a maximum of 100 meters apart at the access points of the river. It is proposed that samples be acquired using clean bottles that can hold a volume of 1.5 litres in 1-week intervals, with sampling time between 8 am and 1 pm on each sampling day. It is recommended that 5 ml of HNO3 be added to each of the samples acquired for preservation. The samples then should be placed in an ice chest with the required capacity of ice to be transported to the university laboratory for analysis.
The proposed study also includes in situ water sampling and quality measurements to obtain the Tano Water Basin's true water quality parameter values. A greater emphasis will be placed on measurements of Total Suspended Matter (TSM) and Chlorophyll-a (Chl-a) concentration, which will be used to determine the Specific Inherent Optical Properties (SIOPs) of the Tano River and Water Basin. It is proposed that the study conduct spectrometer measurements from samples collected in the field to obtain subsurface irradiance reflectance R(0-). These field measures will be adopted from those proposed by Peters et al. (2000).
Furthermore, the water company of Ghana, in addition to the Environmental Protection Agency, will be contacted to acquire 10-year data from 2008 to 2018 on the water quality of Tano River along the designated area to conduct a process capability index analysis. The Ghana Standards will determine the water quality results of chemical determinants such as pH, sulfate, TDS, chloride, iron, manganese, aluminium, total alkalinity, calcium, and fluoride.
Derivation of Chlorophyll-a Concentrations: Chlorophyll Analysis at the Lab
Sampled water will be filtered under a vacuum pump through the Whitemans GF paper of 0.45 μm to retain the pigment after the filtrate is discarded. Chl-a concentrations will be calculated using Eqn. 1 and 2, also known as the Jeffrey and Humphrey Equation. This is to correct for the backscattering of any small particles or fine colloidal matter in the samples (Qin et al., 2007).
Chl aE=11.85Abs 664-1.54(Abs 647)-0.08(Abs 630) 1
Chl as=Chl aE×Extract volume (L)Sample Volume L×Cell Length (m) 2
Chl aE= concentration (mg/L) of chlorophyll a in the extract solution measured,
Abs 664 = sample absorbance at 664 nm (minus absorbance at 750 nm),
Abs 647 = sample absorbance at 647 nm (minus absorbance at 750 nm), and
Abs 630 = sample absorbance at 630 nm (minus absorbance at 750 nm).
Field Spectral Coded Data
With a field view of 250 and approximately 1m above the water surface, field radiometric data measurements will be coded using Interactive Data Language (IDL), which is used to program and retrieve reflected radiance between 400nm and 900nm at an increment of 1.5nm. The raw data will be transformed into MATLAB software to match up the output measurements sampling by an interval of 1nm to produce the water surface spectra and the reflectance measurement.
Calculation of Remote Sensing Reflectance
Remote Sensing Reflectance (Rrs) will be calculated from measured and simulated spectral data using Mobley’s equation:
Rrs=LuEd 3
Lu is the upwelling radiance from the water, and Ed is the downwelling irradiance.
Atmospheric Correction of Satellite Images
The satellite images will be processed for atmospheric and geometric corrections using the Fast Line-of-Sight Atmospheric Analysis of Spectral Hypercubes (FLASH) software package in ENVI5.4. FLASH output is scaled radiance reflectance that equals irradiance reflectance in the case of Lambertian surfaces (Felde et al. 2003). The result of atmospheric correction is the unit less reflectance above the water surface.
Acquisition of Landsat 8 and Sentinel 2 Data
Landsat 8 OLI and Sentinel 2 MSI of the study area will be downloaded from the USGS Earth Explorer Website (http://earthexploere.usgs.gov). The images will be acquired on a date close to the sampling date and ensure they are as cloud-free as possible.
Radiometric Calibration and Geometric Correction
The objective of geometric correction is to ensure that the locations of points in the satellite image match their field locations on the ground. Radiometric calibration consists of equations that convert the TM data's stored quantized energy signal (digital number: DN) into radiance values at the sensor. The Landsat images would be converted into radiance values at the satellite by using the following equation:
Lsens = DN·G+B 4
where
Lsens = Radiance at satellite level of a specific band (W m-2 sr-1 μ -1);
DN = Value of the digital number;
G = Gain and
B = Bias.
The line intercept, described by the bias, considers that even with a null input signal (Lens = 0), the acquisition system can still give an electric output signal fundamental to the acquisition.
Derivation of TSM Concentrations
The proposed study will produce a TSM algorithm using a bio-optical analysis model. The results of the field samples spectrometer will be used for comparative purposes against the laboratory Inherent Optical Properties (IOPs) analysis. A reflectance model will be proposed based on the retrieved data from these optical measures. Remote sensing images will be corrected for atmospheric and air/water interface distortions before applying the TSM algorithm. Following these steps, a radiometric and atmospheric correction is proposed, followed by the R(0-) model applied to the produced images to determine the TSM concentration.
Data Analysis for Assessing Contamination Extent and Variation in Heavy Metals
The data collected will be analyzed using IBM SPSS version 24. The analysis will involve both inferential and descriptive statistics. Descriptive statistics analyzed through SPSS will produce an output of frequencies, measures of central tendency, dispersion, and percentages. The packaged software will also produce an output for an independent sample t-test comparison of average performance to the possibility of statistical differences in physicochemical properties and heavy metals in the Tano River along mine catchments. The proposed research will also use the process capability index to determine the extent to which mineral footprints in the Tano water were within the limits set for water quality in Ghana.
The primary measure used would be the Cpk, as it compares the output of a process to the specification limits using capability indices. The parameter Cp can approximate if the process can produce if the process average is centred between a set limitation. The measure assumes that the process output is normally distributed. While Cpk approximates that the process is capable of production, assuming that the process average is not centred between specific limitations. The measure Cpk can be calculated using the following equation (Mhlongo et al., 2018):
Cpk=USL- μ3σ, μ-LSL3σ
Based on this equation, Cpk is the process capability index, and USL and LSL are the upper and lower limits for quantity under evaluation. The variables are the mean value and standard deviation of the factors.
Conclusion
In the tapestry of water resource dynamics and management, this study has unravelled critical insights into the complex interplay between mining activities and the health of the Tano River and the Water Basin. The exploration of water quality, mineral footprints, and their ecological ramifications underscores the urgency of a comprehensive approach to safeguarding this vital natural resource.
The findings, derived through advanced bio-optical techniques and remote sensing technologies, have illuminated the extent of contamination and variation in heavy metals within the Tano River. Integrating these innovative methodologies has allowed for a nuanced understanding of the intricate relationships that define the water ecosystem, signalling a departure from conventional assessments.
As we navigate the currents of sustainable water management, it is evident that mining operations, particularly in Ghana, demand a recalibration of practices. The study advocates for a paradigm shift in policy frameworks, urging local and national governments to consider the environmental repercussions alongside economic gains. The adverse effects of mining on water quality and ecosystem health necessitate proactive measures, and the study's recommendations serve as a compass for steering towards a more balanced and sustainable future.
In conclusion, the Tano River and Water Basin stand at a crossroads, caught between economic development pressures and the imperative of environmental conservation. This study sheds light on the challenges and paves the way for informed decision-making, presenting a roadmap towards a harmonious coexistence of mining activities and ecological integrity in this crucial watershed.
References
Asamoah, V. E., Asiam, E. K., and Kuma, J. S. (2009). Management of acid mine drainage at Tarkwa, Ghana. In: Yan, E. K., (eds) Appropriate Technologies for Environmental Protection in the Developing World. Dordrecht, Spinger Publications.
Asare-Donkor, N. K., and Adimado, A. A. (2016) Influence of mining-related activities on levels of mercury in water, sediment, and fish from the Ankobra and Tano River basin in South Western Ghana. Environmental Systems Research, 5:5.
Attiogbe, F., and Nkansah, A. (2017). The impact of mining on the water resources in Ghana: Newmont case study at Birim North District (New Abirem). Energy and Environment Research, 7(2), p. 27-36.
Banunle, A., Fei-Baffoe, B., and Otchere, K. G. (2018). Determination of the physicochemical properties and heavy metal status of the Tano River along the Ahafo Mine Catchment in Ghana's Brong-Ahafo region. Journal of Environmental & Analytical Toxicology, 8:3.
Behmel, S., Damour, M., Ludwig, R., and Rodriquex, M. J. (2016). Water quality monitoring strategies – A review and future perspectives. Science of the Total Environment, 571 (15), p.1312-1329.
Gao, L., Bryan, B. A., Liu, J., Li, W., Chen, Y., Liu, R., and Barrett, D. (2017). Managing too little and too much water: Robust mine-water management strategies under variable climate and mine conditions. Journal of Cleaner Production, 162(20), p.1009-1020.
Khan, S., Shahnaz, M., Jehan, N., Rehman, S., Shah, S. R., Shah, M. T., and Din, I. (2013). Drinking water quality and human health risk in Charsadda district, Pakistan. Journal of Cleaner Production, 60(1), 93-101.
Li, H., Yang, Z., Liu, G., Casazza, M., Yin, X. (2017). Analyzing virtual water pollution transfer embodied in economic activities based on grey water footprint: a case study. Journal of Cleaner Production, 161, 1064e1073.
Mhlongo, S., Mativenga, P. T., and Marnewick, A. (2018). Water quality in mining and water-stressed regions. Journal of Cleaner Production, 171, p. 446-456.
NIST/Sematech Engineering Statistics Handbook. What is Process Capability? National Institute of Standards and Technology. Retrieved 2019/7/23. <http://www.itl.nist.gov/div898/handbook/pmc/section1/pmc16.htm.>
Nkhonjera, G.K. (2017). Understanding the impact of climate change on the dwindling water resources of South Africa, focusing mainly on Olifants River basin: a review. Environ. Sci. Policy 71, 19e29.
Northey, S.A., Mudd, G.M., Saarivuori, E. (2016) Water footprinting and mining: where are the limitations and opportunities? J. Clean. Prod. 135, 1098e1116.
Nyantakyi, J. A., Aboagye-Larbi, H., and Acheampong, M. (2013). A comparative assessment of River Tano's upstream and downstream water qualities in Ghana. Journal of Environmental Science and Engineering, p. 283-292.
Owusu-Nimo, F., Mantey, J., Nyarko, K. B., Appiah-Effah, E., and Aubynn, A. (2018). The spatial distribution pattern of illegal small-scale gold mining (galamsey) operations in Ghana focuses on the western region. Heliyon, 4(2), e00534.
Regmi, G., Indraratna, B., Nghiem, L.D. (2009). Long-term performance of a permeable reactive barrier in acid sulfate soil terrain. Water, Air, & Soil Pollut. Focus 9 (5e6), 409e419.
Salem, M.G., Amin, E., (2012). Enhanced dissolved iron and manganese removal from nonconventional water resources in Delta District, Egypt. Energy Procedia, 983e993.
Water Resources Commission. (2012). Tano River Basin- Integrated Water Resources Management Plan. [report] Accra, Ghana.
Yeleliere, E., Cobbina, S. J., and Duwiejuah, A. B. (2018) Review of Ghana’s water resources: the quality and management focusing on freshwater resources. Applied Water Science, 8:93.
Get 3+ Free Dissertation Topics within 24 hours?