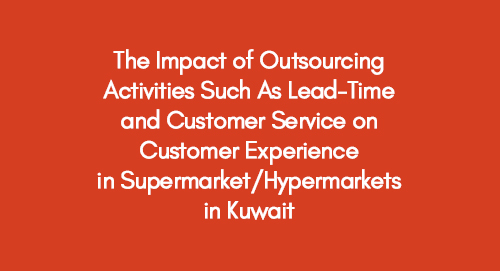
The Impact of Outsourcing Activities Such As Lead-Time and Customer Service on Customer Experience in Supermarket/Hypermarkets in Kuwait
January 18, 2022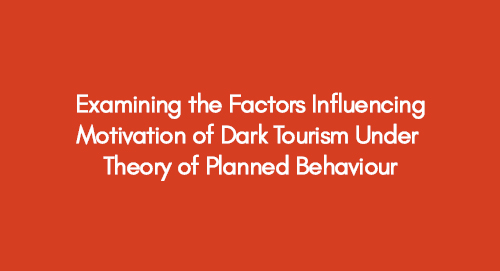
Examining the Factors Influencing Motivation of Dark Tourism Under Theory of Planned Behaviour
January 19, 2022In the Tano River Basin, scientists are using clever techniques to understand the importance of the water quality and minerals it contains. They're using advanced methods like bio-optical modeling and remote sensing to study these aspects from a distance.
Introduction
Researchers are studying the quality of water and the minerals it carries in the Tano River Basin. They're using special tools like bio-optical modeling and remote sensing, which are high-tech ways of looking at the water from far away. By doing this, they can figure out what's in the water and how clean or dirty it is. This helps us understand how healthy the river is and if there are any problems we need to fix to keep the water safe for people and animals.
The following texts are related to back background of the the study.
Contextual Background
Water resources around the globe are experiencing increased stress from urbanization, industrial growth, the proliferation of agricultural activities, and the increasing population. Other attributes of an ever-increasing scarcity of water resources are its exploitation and factors related to global warming. In addition to the dire consequences of scarcity, there is a negative effect of contamination within water catchment areas and water supply systems used to provide clean water.
According to Li et al. (2017), the transfer of contaminants from one body of water to another is a significant issue. These common factors are giving rise to the decline of the quality of water in groundwater, lakes, and even rivers which is becoming a tremendous standing global challenge (Behmel et al., 2016; Khan et al., 2013). Reports from the World Health Organization and UNICEF JMP have asserted that about 2 billion people were using drinking water from a water source contaminated with faeces, with 785 million people lacking a basic drinking water service (WHO, 2017; UNICEF, 2016). The report further states that 144 million people globally collected untreated surface water from lakes, ponds, rivers and streams (WHO, 2017; UNICEF, 2016).
A leading factor of declining water quality is attributed to mining operations (Northey et al., 2016). According to Mhlongo et al. (2017), mining activities are associated with risks related to uncontrolled discharges, flooding of pits, and the collapse of dams noted for water pollution control. Results obtained from Gao et al. (2017) indicate that the mining industry, particularly coal mining, is facing severe challenges related to water resource management, especially avoiding non-compliant discharge of water affected by mining activities.
Execrable water has resulted from traditional mining measures used for water storage infrastructures that are incapable of addressing spill-over with regards to worked water. It has become imperative to research innovative measures that can minimize the risk of unregulated discharges caused by industrial processing or activities. One of the critical steps required is water quality monitoring standardisation according to observations and measurements of the aquatic environment (Behmel et al., 2016).
Discover about Photocatalytic Treatment of WasteWater
Salem and Amin (2012) pointed out that a variety of wastewater activities are known to take place in water catchments which leads to the compromise of biodiversity, food production, human health, and natural ecosystems. Also, Khan et al. (2013) have reported that mining poses a considerable risk towards the access of clean drinking water to the larger populace. It has become essential to investigate the presence and impact of such activities on areas that are water-stressed and in which mining activities take place.
Problem Statement
The mining industry of Ghana is a significant contributor to its economy making up 5 per cent of the country’s GDP, with minerals making 37 per cent of its exports, from which gold contributes 90 per cent of all mineral exports (Ericsson & Lof, 2019). Ghana’s primary focus of the mining and mineral development sector is gold. The main challenge of the industry is its use of acid mine drainage, which expels its pollutants into surface water sources and other water resources. Ghana’s Environmental Protection Agency (EPA), Water Resource Commission (WRC), and Municipal Committees are responsible for the activities and protection of the Tano River and Water Basin.
In 2010 the WRC had issued water use permits for mining and other industrial uses in the basin for a total of 70.3 million m3 per year for both surface and groundwater. A significant issue of the Tano River and its basin is the increased illegal mining activities within the area. Acid mine drainage is defined as wastewater effluent generated from mining operations such as gold (Regmi et al., 2009). This phenomenon is characterized by Regmi et al. (2009) as having low pH content and high heavy mineral content. Acid mine damage is also characterized by high total dissolved solids (TDS), sulphates, and heavy metals. Acid water drainage takes place when mining operations are exposed to oxygen and water, causing a reaction that is catalyzed by bacteria in water-soluble iron sulphates (Mulopo, 2015).
Very little research is available using specialized techniques for assessing the effects of mining in Ghana’s water bodies. A study conducted by Attiogbe and Nkansah (2017) used a mixed-methods model to assess Birim North District water resources to study the physio-chemical parameters (pH, Conductivity, Total Dissolved Solids, Turbidity and Dissolved Oxygen and the concentrations of Arsenic, Lead, Copper, Cadmium and Mercury) for the water bodies comparing it to Ghana’s EPA. However, the primary data of the study was obtained from participants' and non-participants’ observations of mining operations, this meant obtaining data on the physio-chemical properties and perspectives of local residents residing in the area. The study lacks rigorous techniques that may help map out the effects of mining in Ghana’s waterways.
This major gap is attributed to lax data collection and analysis methods that need to be addressed. In their study, Asare-Donkor and Adimado (2016) assessed the mercury levels in the water, sediment and fish from Ankara and Tano River basins. The study was limited by only assessing one measure (mercury, Hg) to reflect on the overall quality of water and its impact on mining-related activities.
This pattern is similar to Macdonald et al. (2015) whose study only measured physicochemical properties from 11 sites along the Surow River, Ghana by focusing on small-scale gold mining operations. The limitation of these studies is their focus on just physicochemical properties at limited sites. The present study proposes to overcome these gaps in methodology by using advanced bio-optical techniques to assess the quality of water by determining the concentration of TSM and chlorophyll-a. The dire impacts of mine wastewater effluent make it critical to research its potential impacts on the Tano River and Tano Water Basin.
Justification of Research
The various residential areas across the Tano River, Ghana, and other communities within the catchment?? area of mines depends on groundwater and surface water for consumption as drink and domestic purposes. Therefore, the lives of humans, flora, and fauna are dependent on the quality of water that runs in the river. Communities that consume contaminated water or aquatic organisms are more prone to hazardous health outcomes.
Other effects of mining activities include pit digging and runoff which becomes a breeding ground for insects such as mosquitoes giving rise to illnesses such as dengue and malaria, and persistent health hazards throughout regions of Ghana and other parts of the African continent. Communities and individuals living close to the Tano River and Water Basin become more exposed to catching these diseases and other water-borne diseases from contaminated sites. There is also a disruption of natural river flow from mining activities that has become a major issue with regard to climate change.
Impact of Environmental Legacies of Geopolitics on Climate Change in Berlin
The adverse effects of mining activities on the Tano River are worth all the attention of researchers. Rigorous research techniques are required to better understand the extent to which mining activities have impacted water bodies to improve policymaking and inject new innovations into the matter. Constant research in this area will aid in improving the quality of water which in turn will have the ability to change the quality of life for human communities, fauna, flora, and the entire environment at large.
Research Aims and Objectives
The primary aim of the current study is to explore and analyze the trends in water quality and mineral footprint along the mining area and water-stressed region along the Tano River and Water Basin. To achieve the aim of the present study the following objectives have been formulated.
- Assess the impact of mining activities on chemical water quality along the Tano River and Water Basin
- Assess the extent of contamination in the surface water of Tano River and Water Basin caused by mining activities in the selected area through physicochemical properties such as pH, turbidity, total dissolved solids (TDS), and electrical conductivity (EC).
- Examine the probable variation in heavy metals such as sulphate, chloride, fluoride, calcium, magnesium, sodium, iron, manganese, and aluminium concentrations in the Tano River that may be caused by mining activities.
- Examine the mining activities' impact on surface water quality along the Tano River Basin acid mine drainage which expels its pollutants into surface water sources – Tano River
- Derive Total Suspended Matter (TSM) and Chlorophyll-a concentrations of Tano River from multi-sensor images and remote sensing techniques.
- Recommend environmental law changes to the local and national government based on the findings of the proposed study.
Study Area
The study area is primarily located between latitudes 6°40' and 7°15' North and Longitudes 2°15' and 2°45' West in Asutifi North District. It shares boundaries with Sunyani Municipal in the North, Tano South District to the North East, Dormaa East District to North West, Asutifi South District to the west, Asunafo North and South Districts in the South West and Ahafo Ano South and North Districts (Ashanti Region) in the South East. With a total land surface area of 1,500 square kilometres, the District is one of the smallest in the Brong Ahafo. The urban forms thirty-two per cent of the District while the rural constitutes seventy-eight per cent. The land size of the Asutifi North District is about 936.31 square kilometres (Nyantakyi, et al., 2013).
The Tano River is a river in Ghana that passes through Cote d’Ivoire (Ivory Coast) and also makes a natural international boundary between Ghana and Cote d’Ivoire before passing into the ocean. The river flows for 400 kilometres into Ehy Lagoon, Tendo Lagoon, and Aby Lagoon in Cote d’Ivoire; then enters the Atlantic Ocean. Tano River can be navigated from its mouth to 95 kilometres to Tanoso; however, travelling further on this route is blocked by Sutre Falls (Asare-Donkor, and Adimado, 2016).
The main watershed of Ghana is attributed to the Tano River and the parallel Ankobra which drains the western portion of a shallow basin that is situated southwest of the Kwahu Plateau. It is noted academics that the Ankobra-Tano Basin is imperative for mining in the country, especially gold and bauxite; while the area is also known for timber, palm oil, rubber, and copra (Owusu-Nimo et al., 2018). Mining activities have caused serious pollution to rivers and streams and have also changed watercourses as a result of excessive siltation (Banunle and Fei-Baffoe, 2018).
Most mining operations in Ghana have increased sedimentation in rivers, especially through the use of hydraulic pumps and suction dredges, which sometimes leave scars on the landscape and as well make the water resources of the country unsafe as a result of the introduction of poisonous chemicals into the river (Banunle and Fei-Baffoe, 2018).
The area under study is the primary river for the Asutifi North District and the Tano River can receive inflows from various streams that drain the Ahafo Mines. The Ahafo mines are divided by seasonal streams and rivers into various basins which include but are not limited to the Amoma basin and Awonsu basins (Banunle et al., 2018). During November towards the end of April, the mentioned basins and their respective tributaries dry up (Asamoah et al., 2009).
During this time, the Tano River remains the most substantial resource of surface water within the designated area. Tano River serves as the main source of water for agricultural and domestic activities. Water resource from the Tano River is pumped and treated by Ghana Water Company Limited (GWCL) at Akyerensua and used to then provide communities in various areas such as New Dormaa, Hwidiem, as well as other communities in the surrounding (Attiogbe and Nkansah, 2017).
The following texts comprise the review of the literature.
Literature Review
Rapid population increase and economic development have imposed plenty of stress on the surface and groundwater systems in many parts of the world, especially in arid and semi-arid areas. The sustainability of water resources in these areas is considered to be an urgent challenge for economic growth. It should be known that the environment must be included as a legitimate user of water resources while ecosystem services have to be considered in assessing economic outputs.
Overview
This goal or vision shift has called for a new approach to water resources management based on the knowledge of interactions among the environment, ecosystems, and economic activities. Many studies have been conducted to improve water resource management which is targeted specifically to maximizing system performance and minimizing environmental stresses by integrating natural processes and human activities into one system of study. The proposed study will review available literature using a thematic review method that organizes available data through comparable themes.
Water Resource Management
International Water Resource Management (IWRM) is inspired by the principles from the Dublin Statement on Water and Sustainable Development made at the International Conference on Water and the Environment (ICWE) in Dublin, in 1992. The first principle states that ―freshwater is a finite and vulnerable resource, essential to sustain life, development, and the environment (ICWE, 1992). This principle demands a holistic approach to management, which identifies all the characteristics of the hydrological cycle and its interaction with other natural resources and ecosystems and considers the demands placed on the resource together with the threats to it.
The base for all IWRM is the hydrological cycle. Rain, the main component of the hydrological cycle, falls on a watershed either intercepted by plants, immersed into the ground or runs over the surface (Chow, 1964). The water that runs overland and joins the stream at some point is the surface runoff while the water that infiltrates into the ground and makes its way into the stream much later is called base flow. How much infiltrates into the ground and how much runs over the surface depends on several different factors, including how porous the ground is, how wet the ground was already, how intense the rainfall is, how arid the area is, how much of the surface is covered by vegetation, how high is the slope in the area and how much of the area is impervious. This interrelationship calls for integrated management between surface and groundwater.
Rivers are always moving and prone to change and both natural and human-induced mechanisms cause rivers to change continuously. Natural changes are gradual and possibly will balance in the long run, while human-induced changes may magnify adverse effects and can imbalance the system rapidly. The degradation of the Mesopotamian marshlands in the Tigris and Euphrates River basins are examples of unsustainable damming and river channelization during the late 1980s (UNEP, 2001).
Further, land-use change brought by deforestation and urbanization alters rates of erosion, infiltration, and overland flow. High rates of erosion are in turn responsible for reducing the capacity of reservoirs of dams constructed for hydropower and irrigation and inducing flooding downstream (Flintan and Tamrat, 2002). Climate change is also induced by land-use change, which has impacts on both water quantity and quality (IPCC, 2000). The increment of rainfall intensity causes detachment of soils on degraded and bare land that increases sediment transport and non-point sources pollutants to the streams; high surface runoff that washes out wastes and garbage, especially in urban areas with poor drainage systems, to the streams; and the increasing carbon dioxide content in the air that affects the acidity of rainwater are some of the examples.
Flood Risk and Flood Defence Management in Times of Climate Change
The interaction shows that the hydrologic cycle goes through various complicated processes using air, soil, vegetation, surface, and groundwater as media. Hence, the integration of land and water management is indispensable to account for all the interactions and in managing the relationships between quantity and quality and upstream and downstream water interests (GWP, 2000).
According to GWP (2000), the implementation of IWRM would come as a possibility when helped by management instruments, which are tools that enable and help decision-makers to make coherent choices between different alternatives. Among the methods, water resources assessments and the development of its knowledge base are necessary for effective water management. To evaluate the resource availability and quality against the demands, the assessment should address the occurrence in space and time of both surface and groundwater. Likewise, Irvine et al. (2005) stated that effective implementation of the WFD requires the utilization of mathematical models, to provide a synthesis of complex natural processes and to identify the likely response within and among domains of natural and anthropogenic changes.
Here is the methodology of the study below.
Methodology
The data collected for the present study is proposed to be acquired from the municipality and Ghana water authority data from the Asutifi North District and the streams that drain the Ahafo Mines. A total of thirty (30) samples are proposed to be taken with duplicates from access points to the Tano River in the Asutifi North District.
Water Sampling and Treatment
The proposed sample size is recommended by Asare-Donkor and Adimado, (2016); Mhlongo et al. (2018); and Attiogbe and Nkansah, (2017) who argue that thirty samples are composed of duplicate collection from both upstream and downstream access points on the Tano River. It is proposed that samples be obtained at a maximum of 100 meters apart at the access points of the river. It is proposed that samples be acquired using clean bottles that can hold a volume of 1.5 liters in a 1-week interval, with sampling time between 8 am and 1 pm on each sampling day. It is recommended that 5 ml of HNO3 be added to each of the samples acquired for preservation. The samples then should be placed in an ice chest with the required capacity of ice to be transported to the university laboratory for analysis.
The proposed study also includes in situ water sampling and quality measurements to obtain the true water quality parameter values of the Tano Water Basin. A greater emphasis will be placed on measurements of Total Suspended Matter (TSM) and Chlorophyll-a (Chl-a) concentration which will be used to determine the Specific Inherent Optical Properties (SIOPs) of the Tano River and Water Basin. It is proposed that the study conduct spectrometer measurements from samples collected in the field to obtain subsurface irradiance reflectance R(0-). These field measures will be adopted from the measures proposed by Peters et al. (2000).
Furthermore, the water company of Ghana in addition to the Environmental Protection Agency will be contacted to acquire 10-year data from 2008 to 2018 on the water quality of Tano River along the designated area to conduct a process capability index analysis. The Ghana Standards will be used to determine the water quality results of chemical determinants such as pH, sulfate, TDS, chloride, iron, manganese, aluminum, total alkalinity, calcium, and fluoride.
Derivation of Chlorophyll-a Concentrations
Researchers are figuring out the amount of chlorophyll-a in the water, which is like a green pigment that helps plants make food through photosynthesis. They're using special techniques to calculate these concentrations, which can tell us a lot about the health of aquatic ecosystems.
1. Chlorophyll Analysis at the Lab
Sampled water will be filtered under a vacuum pump through the Whitemans GF paper of 0.45 μm to retain the pigment after the filtrate is discarded. Chl-a concentrations will be calculated using Eqn. 1 and 2 which is also known as the Jeffrey and Humphrey Equation. This is to correct for the backscattering of any small particles or fine colloidal matter in the samples (Qin et al., 2007).
= concentration (mg/L) of chlorophyll a in the extract solution measured,
Abs 664 = sample absorbance at 664 nm (minus absorbance at 750 nm),
Abs 647 = sample absorbance at 647 nm (minus absorbance at 750 nm), and
Abs 630 = sample absorbance at 630 nm (minus absorbance at 750 nm).
2. Field Spectral Coded Data
With a field view of 250 and positioned approximately 1m above the water surface, field radiometric data measurements will be coded using Interactive Data Language (IDL), which is used to program and retrieve reflected radiance between 400nm and 900nm at an increment of 1.5nm. The raw data will be transformed into MATLAB software, so it can match up the output measurements sampling by the interval of 1nm to produce the water surface spectra and the reflectance measurement.
3. Calculation of Remote Sensing Reflectance
Remote Sensing Reflectance (Rrs) will be calculated from measured and simulated spectral data using Mobley’s equation:
where Lu is the upwelling radiance from the water, and Ed is the downwelling irradiance.
4. Atmospheric Correction of Satellite Images
The satellite images will be processed for atmospheric and geometric corrections using the Fast Line-of-Sight Atmospheric Analysis of Spectral Hypercubes (FLASH) software package in ENVI5.4. FLASH output is scaled radiance reflectance that equals irradiance reflectance in the case of Lambertian surfaces (Felde et al. 2003). The result of atmospheric correction is the unit less reflectance above the water surface.
5. Acquisition of Landsat 8 and Sentinel 2 data
Both Landsat 8 OLI and Sentinel 2 MSI of the study area will be downloaded from the USGS Earth Explorer Website (http://earthexploere.usgs.gov). The images will be acquired on a date that is close to the sampling date and ensure that they are as cloud-free as possible.
6. Radiometric Calibration and Geometric Correction
The objective of geometric correction is to ensure that the locations of points in the satellite image match their field locations on the ground. Radiometric calibration consists of a series of equations used to convert the stored quantized energy signal (digital number: DN) of the TM data into radiance values at the sensor. The Landsat images would be converted into radiance values at the satellite by using the following equation:
Lsens = DN·G+B
where;
Lsens = Radiance at satellite level of a specific band (W m-2 sr-1 μ -1);
DN = Value of the digital number;
G = Gain and
B = Bias.
The line intercept, described by the bias, takes into account the fact that even with a null input signal (Lsens = 0) the acquisition system can still give an electric output signal fundamental to the acquisition
Derivation of TSM Concentrations
The proposed study will produce a TSM algorithm that will use a bio-optical model of analysis. The results of the field samples spectrometer will be used for comparative purposes against the laboratory Inherent Optical Properties (IOPs) analysis. Based on the retrieved data from these optical measures a reflectance model will be proposed. Remote sensing images will be corrected for atmospheric and air/water interface distortions before the TSM algorithm is applied. Following these steps, a radiometric and atmospheric correction is proposed, followed by the R(0-) model application to produce images that determine the TSM concentration.
1. Data Analysis to Assess the Extent of Contamination and Variation in Heavy Metals
The data collected will be analyzed using IBM SPSS version 24. The analysis will involve both inferential and descriptive statistics. Descriptive statistics analyzed through SPSS will produce an output of frequencies, measures of central tendency, dispersion, and percentages. The packaged software will also be used to produce an output for an independent sample t-test comparison of average performance to the possibility of statistical differences in physicochemical properties and heavy metals in the Tano River along mine catchments.
The data that is obtained from experimentation is compared to the data that is obtained from EPA standards already set out. These government documentations already mention average desired numerals for physicochemical properties and average permissible heavy metals rates. The purpose of the t-tests is to compare the experimental study with the secondary obtained material. Akin to this comparison, the study also looks to produce its capability index for the properties being analyzed. In short, there is a comparison of contamination that takes place for the samples that are obtained. The proposed research will also use the process capability index to determine the extent to which mineral footprints in the Tano water were within the limits set for water quality in Ghana.
The primary measure used would be the Cpk as it compares the output of a process to the specification limits by using capability indices. The parameter Cp can approximate if the process can produce if the process average is centered between a set limitation. The measure assumes that the process output is normally distributed. While Cpk approximates that the process is capable of production assuming that the process average is not centered between specific limitations. The measure Cpk can be calculated using the following equation (Mhlongo et al., 2018):
Based on this equation, Cpk is the process capability index, and USL and LSL are the upper and lower limits for quantity under evaluation respectively. The variables are the mean value and standard deviation of the factors.
Conclusion
In conclusion, the study reveals valuable insights into the water quality and mineral content of the Tano River Basin. The application of bio-optical modelling and remote sensing techniques has proven instrumental in understanding and monitoring the health of the river ecosystem. These findings underscore the importance of continued research and proactive measures to safeguard the water resources in the Tano River Basin.
Below are the references cited in the study.
Reference
Asamoah, V. E., Asiam, E. K., and Kuma, J. S. (2009). Management of acid mine drainage at Tarkwa, Ghana. In: Yan, E. K., (eds) Appropriate Technologies for Environmental Protection in the Developing World. Dordrecht, Springer Publications.
Asare-Donkor, N. K., and Adimado, A. A. (2016) Influence of mining-related activities on levels of mercury in water, sediment and fish from the Ankobra and Tano River basin in South Western Ghana. Environmental Systems Research, 5:5.
Attiogbe, F., and Nkansah, A. (2017). The impact of mining on the water resources in Ghana: Newmont case study at Birim North District (New Abirem). Energy and Environment Research, 7(2), p. 27-36.
Banunle, A., Fei-Baffoe, B., and Otchere, K. G. (2018). Determination of the physicochemical properties and heavy metal status of Tano River along the Catchment of the Ahafo Mine in the Brong-Ahafo region of Ghana. Journal of Environmental & Analytical Toxicology, 8:3.
Behmel, S., Damour, M., Ludwig, R., and Rodriquex, M. J. (2016). Water quality monitoring strategies – A review and future perspectives. Science of the Total Environment, 571 (15), p.1312-1329.
Gao, L., Bryan, B. A., Liu, J., Li, W., Chen, Y., Liu, R., and Barrett, D. (2017). Managing too little and too much water: Robust mine-water management strategies under variable climate and mine conditions. Journal of Cleaner Production, 162(20), p.1009-1020.
Khan, S., Shahnaz, M., Jehan, N., Rehman, S., Shah, S. R., Shah, M. T., and Din, I. (2013). Drinking water quality and human health risk in Charsadda district, Pakistan. Journal of Cleaner Production, 60(1), 93-101.
Li, H., Yang, Z., Liu, G., Casazza, M., Yin, X. (2017). Analyzing virtual water pollution transfer embodied in economic activities based on greywater footprint: a case study. Journal of Cleaner Production, 161, 1064e1073.
Mhlongo, S., Mativenga, P. T., and Marnewick, A. (2018). Water quality in mining and water-stressed region. Journal of Cleaner Production, 171, p. 446-456.
NIST/Sematech Engineering Statistics Handbook. What is Process Capability? National Institute of Standards and Technology. Retrieved 2019/7/23. <http://www.itl.nist.gov/div898/handbook/pmc/section1/pmc16.htm.>
Nkhonjera, G.K. (2017). Understanding the impact of climate change on the dwindling water resources of South Africa, focusing mainly on the Olifants River basin: a review. Environ. Sci. Policy 71, 19e29.
Northey, S.A., Mudd, G.M., Saarivuori, E. (2016) Water footprinting and mining: where are the limitations and opportunities? J. Clean. Prod. 135, 1098e1116.
Nyantakyi, J. A., Aboagye-Larbi, H., and Acheampong, M. (2013). A comparative assessment of the upstream and downstream water qualities of River Tano in Ghana. Journal of Environmental Science and Engineering, p. 283-292.
Owusu-Nimo, F., Mantey, J., Nyarko, K. B., Appiah-Effah, E., and Aubynn, A. (2018). Spatial distribution pattern of illegal small scale gold mining (galamsey) operations in Ghana: a focus on the western region. Heliyon, 4(2), e00534.
Regmi, G., Indraratna, B., Nghiem, L.D. (2009). Long-term performance of a permeable reactive barrier in acid sulphate soil terrain. Water, Air, & Soil Pollut. Focus 9 (5e6), 409e419.
Salem, M.G., Amin, E., (2012). Enhanced removal of dissolved iron and manganese from nonconventional water resources in Delta District, Egypt. Energy Procedia, 983e993.
Water Resources Commission. (2012). Tano River Basin- Integrated Water Resources Management Plan. [report] Accra, Ghana.
Yeleliere, E., Cobbina, S. J., and Duwiejuah, A. B. (2018) Review of Ghana’s water resources: the quality and management with particular focus on freshwater resources. Applied Water Science, 8:93.
Get 3+ Free Dissertation Topics within 24 hours?