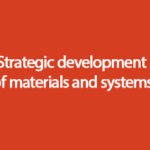
Material Performativity for Biological Growth
January 28, 2021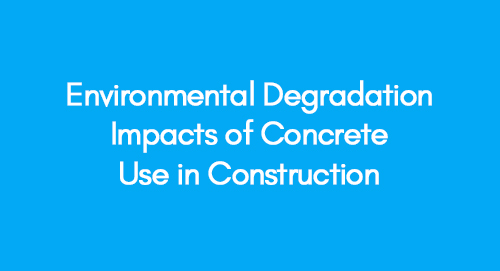
Environmental Degradation Impacts of Concrete Use in Construction
January 28, 2021In the alchemy of environmental stewardship, soil remediation technologies transmute contamination into rejuvenation, where innovation becomes the green thumb nurturing the Earth's hidden vitality.
Outline
- Introduction
- Human Impacts on the Environment
- Sources of Ground Pollution
- Soil Remediation Technologies
- Discussions
- Conclusion
- References
The well-being of our planet hinges on the resilience of its ecosystems, with soil standing as a pivotal element in this intricate equilibrium. Regrettably, the repercussions of industrialization, inadequate waste management, and various human interventions have left numerous regions across the globe grappling with soil contamination. Amidst this environmental challenge, soil remediation technologies have surfaced as a promising ray of hope, introducing inventive solutions to rejuvenate and preserve the Earth's soil health. In this blog, we embark on a journey to unravel the diverse soil remediation technologies that are actively contributing to the restoration of our environment.
Explore More About Soil Remediation Technologies
From the relentless march of industrial progress to the careless disposal of waste, human activities have taken a toll on the health of our soil. As a result, a pressing need for remediation has arisen, prompting the development of cutting-edge technologies designed to counteract the adverse effects of contamination. In the pages that follow, we delve into the arsenal of soil remediation technologies that hold the potential to mitigate the impacts of human-induced soil degradation, offering a glimpse into the positive transformations underway in our efforts to safeguard the Earth's vital ecosystems.
Introduction
Industrial and social mishaps are primary contributors to environmental pollution, marked by incidents such as leaks in waste disposal, spills during transportation, and leakages in industrial storage facilities. Petroleum-contaminated sites, in particular, pose significant challenges for cleanup, necessitating the development of advanced soil and water remediation technologies. The urgency lies in crafting remediation solutions that are swift, practical, and adaptable across a spectrum of physical environments where various ongoing processes occur. Both the public and governmental bodies in the industrial sector recognize the potential hazards posed by chemical constituents like PCBs (polychloro biphenyls), TPH (total petroleum hydrocarbons), heavy metals, pesticides, and PAHs (polycyclic aromatic hydrocarbons), directly impacting the environment and human health.
Numerous remediation technologies have been innovated to address environmental contaminations, targeting pollutants in soil, wastewater, leachate, and groundwater (Riser-Roberts, 1998). A strategic amalgamation of remediation technologies becomes essential in contaminated soil to achieve optimal conditions tailored to specific circumstances. When applied synergistically, combining chemical, physical, and biological remediation technologies can reduce contamination to acceptable and safe levels (Reddy et al., 1999; RAAG, 2000). This report outlines various soil remediation technologies and assesses human impacts on the environment, examining the sources of ground pollution attributable to human activities.
The discussion encompasses the technology's applicability, associated concerns, processes, limitations, advantages, and site-specific parameters. The choice of a particular remediation technology hinges on factors such as site characteristics, costs, regulatory requirements, and project time constraints (Riser-Roberts, 1998; Reddy et al., 1999). Selecting an appropriate technology is a crucial step towards successfully remedying contaminated sites. The treatment of contaminated soils relies on meticulous design selection, adaptable to varying contaminants and soil properties, ensuring the optimal performance of the applied system (Chareneau et al., 1995; API, 1993; Alexander, 2003; Demars et al., 1995; Anderson 1995; USEPA, 1995–1998; Reddy et al., 1999; RAAG, 2000; Feng et al., 2001; Hejazi, 2002; Kao et al., 2001; Li et al., 2002; Abbott et al., 2002; Guerin et al., 2002; Barnes, 2003; Chu, 2003; Halmemies et al., 2003; Khan and Husain, 2003; Juhasz et al., 2003; Liang et al., 2003; Wilk, 2003; Wait and Thomas, 2003).
Human Impacts on the Environment
In recent decades, significant technological strides have substantially benefited human communities. Engineered materials and inventions have enhanced the quality of life and contributed to an improved human survival rate. These technological advancements enable humans to thrive in diverse and challenging environments. However, amid these developments, the environmental impact of human-engineered materials and inventions on both biotic and abiotic elements has often been overlooked.
As human communities continue to flourish, their consumption of essential resources like water, food, and oxygen has increased, accompanied by the generation of substantial amounts of waste. Using energy for cooling, transportation, heating, and industrial operations is integral to modern living. In the quest for survival, humans interact with the environment's living (biotic) and non-living (abiotic) components, forging a delicate balance on Earth.
Despite the undeniable benefits of technological progress, there is a growing concern regarding the inadvertent environmental consequences associated with its development. The production of large quantities of waste stemming from various human activities poses a significant challenge. While some of this waste is organic and can be reintegrated into the soil through recycling procedures, the scale and complexity of waste production often exceed sustainable management practices.
In some instances, communities opt for the disposal of waste in landfills, which, under certain conditions, can lead to environmental hazards. Landfill sites have been reported to leak, introducing toxic chemicals into the surrounding soil and water. These pollutants, in turn, have been linked to detrimental effects on living organisms, contributing to ecological imbalances and, in extreme cases, causing harm or death to various species.
The undeniable reality is that human activities have left an indelible mark on the environment, impacting both biotic and abiotic elements. As we navigate the complexities of technological advancement, adopting a holistic approach that considers the long-term ecological consequences of our actions becomes imperative. Balancing progress with environmental stewardship is key to ensuring a sustainable and harmonious coexistence between human communities and the intricate web of life on Earth.
Air
The considerable pollution of the air is primarily attributed to human activities involving the transportation, heating, and processing of fossil fuels and industrial substances. These actions result in substantial emissions of greenhouse gases, consequently contributing to the depletion of the ozone layer.
Water
Human activities, marked by high consumption, have notably impacted water levels. The development of human communities has altered the flow of water through natural watersheds. Furthermore, water quality is compromised due to the careless and improper disposal of industrial chemicals, leading to chemical spills that significantly contaminate oceans, lakes, rivers, and underground water sources.
Habitat
Human activities have disrupted natural habitats, resulting in a decline in the number of producers within food chains. This has led to the extinction of numerous species, causing significant disturbances to ecosystems.
Figure 1: soil contamination and effects on water quality
Sources of Ground Pollution
The primary contributors to land pollution encompass industrial factories, human sewage, nuclear and chemical plants, oil refineries, littering, landfills, construction debris, mining, and oil spills.
Land pollution is predominantly a consequence of urbanization, which consumes forested areas. The demand for raw materials has led to the destruction and exploitation of forests. The construction of reservoirs, driven by a heightened need for water, further diminishes available land. Increased demand for food has expanded agricultural land at the expense of forests. Daily, tons of domestic waste are indiscriminately dumped, posing potentially devastating impacts on groundwater and soil when not disposed of properly. Although incinerators are commonly used to treat waste, a significant amount remains unburnt, contributing to the proliferation of dumping grounds. This practice has elevated the volume of solid waste, adversely affecting groundwater and soil quality.
The escalating volume of waste necessitates the discovery of new dumping grounds. Using pesticides and herbicides to enhance crop yield has introduced pollutants into the soil and water bodies. Industrial activities further exacerbate land pollution, particularly through open-cast mining, which creates deep pools with hazardous depths. The soil is contaminated by mining wastes that often contain various toxic substances.
Soil Remediation Technologies
Researchers and practitioners have innovated a variety of soil remediation technologies, and this section will delve into a chosen set of these innovative approaches:
Soil Washing
Water and various solvents are combined to wash soils, followed by scrubbing processes using different mechanical equipment. To dissolve specific contaminants, solvents are carefully selected and evaluated for their health and environmental impacts (Asante-Duah, 1996; Feng et al., 2001; Chu and Chan, 2003; Urum et al., 2003). The soil-washing process typically segregates coarse soil from fine soil. Hydrocarbon contaminants often sorb and bind to smaller soil particles, facilitating the separation of larger particles thereby reducing the volume of contaminated soil (Riser-Roberts, 1998).
The smaller soil volume, containing most clay and silt particles, can undergo additional treatment methods such as bioremediation and incineration for further soil remediation. The larger soil volume, considered clean, can be repurposed for backfilling (USEPA, 1996a; RAAG, 2000; Chu and Chan, 2003). Combining soil washing with other technologies enhances the efficiency of soil decontamination.
Soil Vapour Extraction
Vacuum extraction, also known as soil venting or soil vapour extraction, stands as a widely accepted, cost-effective soil remediation technology acknowledged for its effectiveness, particularly in soils heavily contaminated by SVOCs and VOCs (Suthersan, 1997; Zhan and Park, 2002; Halmemies et al., 2003). In this technique, horizontal or vertical wells are installed in the contaminated soil area, facilitating evaporation through air blowers. Vacuums applied near the contamination source, through wells, aid in evaporating volatile components from the contaminated mass.
Extraction wells are then utilized to remove the evaporated components permanently. Carbon adsorption methods treat the extracted vapours before their release into the atmosphere (USEPA, 1995a). Soil vapour extraction also stimulates the biodegradation of less volatile contaminants through provided subsurfaces (USEPA, 1996b, 1998a; Halmemies et al., 2003; Harper et al., 2003). This technique can be applied not only for soil treatment but also for the remediation of contaminated groundwater and air stripping. Figure 2 provides a simplified illustration of the soil remediation technique.
Figure 2: soil vapour extraction process
Chemical Extraction
The chemical extraction process, also known as the hazardous component separation process, involves the removal of harmful elements from the soil. Unlike the simple soil-washing process that utilizes only water or water additives, the chemical extraction process uses specific chemicals. This technique is not designed to destroy waste found in sludges, soils, and sediments but aims to reduce the soil volume as part of the remediation procedure. The effectiveness of the process can vary based on the equipment used, the chemical employed, and the type of operation considered (Barnes, 2003).
Figure 3: chemical extraction
Before chemical extraction remediation, the soil is typically divided into fine and coarse fractions through physical separation steps. A common assumption is that the fine portion contains a higher concentration of contaminants. The effectiveness of the extraction kinetics can be improved through physical separation processes that isolate heavy metals and related particulates from the soil.
Acid Extraction
Another effective decontaminating agent for soil is acid. Hydrochloric acid is commonly utilized to remove contaminants, particularly heavy metals, from the soil. The initial step involves the removal of coarse solids from the soil through physical screening procedures. In the extraction unit, hydrochloric acid is introduced to the contaminated soil. The residence time of the process varies based on factors such as soil type, contaminant concentration, and specific contaminants for a given unit.
The continuous pumping of the mixture of the extracting element (acid) and soil occurs from the mixing tank. Hydro-cyclones are employed to separate the extracting element and the soil. After the extraction process, the soils are transported to the rinse system. Rinsed water removes metals and acids, with commercially available precipitants like sodium hydroxide and lime, along with flocculants, aiding in removing metals and acid reformation (Abbott et al., 2002). The concentrated metals retrieved from this process hold the potential for recovery. Subsequently, water is extracted from the soils, and any remaining acid is neutralized by blending it with fertilizers or lime.
Solvent Extraction
A prevalent form of chemical extraction involves solvent extraction, utilizing organic solvents as the extracting agents. This technique is increasingly integrated with other technologies such as stabilization, solidification, soil washing, and incineration. In certain cases, the technology is employed as an independent remediation process. Its efficacy lies in removing target contaminants, particularly metals bound organically with the soil.
Despite its success, the treated soil matrix may retain traces of the solvent, necessitating a thorough consideration of solvent toxicity. The mixture is reintroduced to the site following treatment with the best available technology. The chemical extraction technology in soil remediation typically requires medium-term maintenance and operation.
Land Disposal
Another method of waste treatment is known as landfilling. The construction of double-liners around landfills is mandated to ensure the safe introduction of hazardous contaminants from offsite locations. These liners facilitate release response programs, leachate collection, systems to detect leakage, and groundwater monitoring (Barnes, 2003). The acceptance criteria for various wastes restrict the types disposed of in landfills. Landfill remedial units are applied to onsite remedial actions and generators.
Meeting the standards and permitted limits is crucial, with adjustments in size or requirements based on specific waste or site conditions (Halmemies et al., 2003). It is preferable to leave disposal units in locations compliant with predefined standards and regulations outlined in waste disposal policies established before their promulgation.
Figure 4: A typical landfill
Incineration
Organic contaminants present in the soil can undergo combustion at elevated temperatures, typically ranging from 870 to 1,200 °C in the presence of oxygen. When exposed to oxygen, refractory and halogenated contaminants are incinerated and volatilized at these high temperatures. The combustion process is typically sustained and initiated using auxiliary fuels. Incinerators exhibit high efficiency in destroying and removing contaminants, often reaching 99.99%. Notably, for substances like PCBs and dioxins, efficiencies exceeding 99.9999% have been observed (Halmemies et al., 2003). However, further treatment is necessary for the combustion residuals and off-gases.
Figure 5: incineration
Discussions
Advancements in remediation technologies are crucial for accelerating aquifer cleanups and improving soil treatments. In soil and groundwater, multi-phase plumes with diverse movement directions pose challenges, as contaminants are often difficult to locate, allowing even small amounts to impact larger areas. The efficiency of treatment processes relies on factors such as contaminant sources and the time required for soil and water remediation. Contaminant discharge from the source zone can have both short and long-term impacts, emphasizing the need for comprehensive risk management strategies. Persistent contaminants like sediment underscores and PCBs in soil necessitate ecological indicators for risk assessment.
Biological indicators play a crucial role in assessing ecosystem conditions and the adverse effects of contaminants. Remediation of contaminated sediments requires effective indicators and risk management strategies (Wait and Thomas, 2003). Over the past decade, useful indication and monitoring techniques have been developed for controlling and identifying contaminants. Evaluating procedures and natural recovery techniques assess the performance of extractive elements. Continuous development of in situ remediation techniques is essential for challenging geological formations.
Effective technologies have been developed for remediating soil contaminated with metals, increasing the demand for in situ procedures. Establishing standards for industrial and residential emissions into groundwater and soil is essential. Remediation procedures must process soil contaminants to protect waterways and other groundwater bodies. Comparing natural background levels with standards used in recent remediation techniques allows for adjustments to new standards.
Adjusting residential standards near industrial zones ensures compliance monitoring against all standards. Monitoring compliance is critical; any detected emissions should be reported to local communities or relevant authorities. Enforcing penalties for individuals responsible for pollution, including potential jail sentences, is important for continuous and significant breaches. While continuous breaches are not preferable, compliance should be considered a normal expense for industries or businesses. Establishing significant penalties for polluters encourages awareness of environmental safety standards.
Conclusion
References
- Asante-Duah, D.K., 1996. Managing Contaminated Sites: Problem Diagnosis and Development of Site Restoration, Wiley, New York, NY
- Abbott, E.H., Bell, J.T., Fjeld, R.A., Peterson, J.R., Sivavec, T.M., 2002. In situ Treatment of Mercury Contaminated Soils, Center for Research and Technology Development, (Publication) CRTD, American Society of Mechanical Engineers, vol. 64, pp. 769–773
- API, 1993, Guide for Assessing and Remediating Petroleum Hydrocarbons in Soils, American Petroleum Institute, API publication number 1629, Washington, DC.
- Anderson, W.C., 1995. Innovative Site Remediation Technologies, vol. 1–8. American Academy of Environmental Engineers, Annapolis, ML
- Alexander, M., 1994, Biodegradation and Bioremediation, Academic Press, San Diego, CA
- Barnes, D.L., Cosden, E., Johnson, B., Johnson, K., Stjarnstrom, S., Johansson, K., Filler, D., 2002, Operation of Soil Vapor Extraction in Cold Climates, Cold Regions Engineering Cold Regions Impacts on Transportation and Infrastructure, Proceedings of the Eleventh International Conference, May 20–22 2002, Anchorage, AK, pp. 956–967.
- Chu, W., 2003, Remediation of contaminated soils by surfactant-aided soil washing, Practice Periodical of Hazardous, Toxic, and Radioactive Waste Management 7(1), pp 19–24
- Chu, W., Chan, K.H., 2003, The mechanism of the surfactant-aided soil washing system for hydrophobic and partial hydrophobic organics, Science of the Total Environment 307(1–3), pp 83–92
- Chareneau, R.J., Bedient, P.B., Loehr, R.C., 1995. Groundwater Remediation, vol. 8, Technomic Publication Company, Lancaster, PA
- Demars, K.R., Richardson, G.N., Yond, R.N., Chaney R.C., 1995, Dredging, remediation, and containment of contaminated sediments, ASTM Publication No, STP 1293, American Society of Testing Materials, Philadelphia, PA
- Feng, D., Lorenzen, L., Aldrich, C., Mare, P.W., 2001. Ex-situ diesel-contaminated soil washing with mechanical methods. Minerals Engineering 14(9), pp 1093–1100
- Guerin, T.F., Horner, S., McGovern, T., Davey, B., 2002. An application of permeable reactive barrier technology to petroleum hydrocarbon contaminated groundwater. Water Research 36(1), pp 15–24.
- Hejazi, R.F., 2002. Oily Sludge Degradation Study Under Arid Conditions Using a Combination of Landfarm and Bioreactor Technologies. PhD thesis, Faculty of Engineering and Applied Science, Memorial University of Newfoundland, St John’s, Canada
- Kao, C.M., Chen, S.C., Liu, J.K., Wu, M.J., 2001, Evaluation of TCDD biodegradability under different redox conditions, Chemosphere 44(6), pp 1447–1454.
- Juhasz, A.L., Smith, E., Smith, J., Naidu, R., 2003b. In situ remediation of DDT-contaminated soil using a two-phase co-solvent flushingfungal biosorption process, Water, Air, and Soil Pollution 147(1–4), pp 263–274.
- Halmemies, S., Grondahl, S., Arffman, M., Nenonen, K., Tuhkanen, T., 2003. Vacuum extraction-based response equipment for recovery of fresh fuel spills from soil. Journal of Hazardous Materials 97(1–4), pp 127–143
- Khan, F.I., Husain, T., 2003, Evaluation of a petroleum hydrocarbon contaminated site for natural attenuation using ‘RBMNA’ methodology, Environmental Modeling and Software 18, pp179–194.
- Liang, S., Min, J.H., Davis, M.K., Green, J.F., Remer, D.S., 2003, Use of pulsed-UV processes to destroy NDMA, American Water Works Association 95(9), pp 121–131,
- Riser-Roberts, E., (1998), Remediation of Petroleum Contaminated Soil: Biological, Physical, and Chemical Processes, Lewis Publishers, Boca Raton, FL
- Reddy, K.R., Admas, J.F., Richardson, C., 1999, Potential technologies for remediation of Brownfield, Practice Periodical of Hazardous, Toxic, and Radioactive Waste Management 3(2), pp 61–68
- RAAG, 2000, Evaluation of Risk Based Corrective Action Model, Remediation Alternative Assessment Group, Memorial University of Newfoundland, St John’s, NF, Canada
- Suthersan, S.S., 1997. Remediation Engineering: Design Concepts, Lewis Publishers, Boca Raton, FL
- USEPA, 1995a, How to Evaluate alternative cleanup technologies for underground storage tank sites, Office of Solid Waste and Emergency Response, US Environmental Protection Agency, Publication # EPA 510-B-95-007, Washington, DC
- USEPA, 1995b, Abstracts of remediation case studies, Office of Solid Waste and Emergency Response, US Environmental Protection Agency, Publication number EPA-542-R-95-001, Washington, DC
- USEPA, 1996a, A citizen’s guide to soil washing. Office of Solid Waste and Emergency Response, US Environmental Protection Agency Publication # EPA 542-F-96-002, Washington, DC
- USEPA, 1996c, A citizen’s guide to natural attenuation, Office of Solid Waste and Emergency Response, US Environmental Protection Agency, Publication # EPA 542-F-96-015, Washington, DC
- USEPA, 1996d, A citizen’s guide to treatment walls, Office of Solid Waste and Emergency Response, US Environmental Protection Agency. Publication # EPA 542-F-96-016, Washington, DC
- USEPA, 1996e, A citizen’s guide to in situ soil flushing. Office of Solid Waste and Emergency Response, US Environmental Protection Agency, Publication # EPA 542-F-96-006, Washington, DC
- USEPA, 1996f, A citizen’s guide to in situ thermal desorption. Office of Solid Waste and Emergency Response, US Environmental Protection Agency, Publication # EPA 542-F-96-005, Washington, DC
- USEPA, 1996g, A citizen’s guide to phytoremediation. Office of Solid Waste and Emergency Response, US Environmental Protection Agency, Publication # EPA 542-F-96-014, Washington, DC
- USEPA, 1996h, A citizen’s guide to bioremediation, Office of Solid Waste and Emergency Response, US Environmental Protection Agency, Publication # EPA 542-F-96-007, Washington, DC
- USEPA, 1998a, Soil vapour extraction (SVE), Office of the Underground Storage Tank, US Environmental Protection Agency, Publication # EPA 510-B-95-007, [available online at] http://www.epa.gov/swerust1/cat/sve1.htm, retrieved 19th February 2013
- USEPA, 1998d, Office of the Underground Storage Tank, USEnvironmental Protection Agency, Publication # EPA 510-B-95-007, [available online at], http://www.epa.gov/OUST/cat/biovent.htm retrieved 19th February, 2013
- USEPA, 1998e, Office of the Underground Storage Tank, US Environmental Protection Agency, Publication # EPA 510-B-95-007, [available online at] http://www.epa.gov/swerust1/cat/biopiles.htm retrieved 19th February 2013
- USEPA, 1998f, Field Application of in situ remediation technologies: ground-water circulation wells. US Environmental Protection Agency, Publication # EPA 542-R-98-009, Washington, DC
- Urum, K., Pekdemir, T., Gopur, M., 2003, Optimum conditions for washing of crude oil-contaminated soil with biosurfactant solutions, Process Safety and Environmental Protection: Transactions of the Institution of Chemical Engineers, Part B 81(3), pp 203–209
- Wilk, C.M., 2003. Solidification/stabilization treatment: principles and practice. Air and Waste Management Association’s Magazine for Environmental Managers July, pp 31–37
- Wait, S.T., Thomas, D., 2003. The Characterization of Base Oil Recovered From the Low-Temperature Thermal Desorption of Drill Cuttings, SPE/EPA Exploration and Production Environmental Conference, Mar 10–12, San Antonio, TX, pp. 151–158.
- Zhan, H., Park, E., 2002. Vapour flow to horizontal wells in unsaturated zones. Soil Science Society of America Journal 66(3), pp 710–721.
Get 3+ Free Dissertation Topics within 24 hours?