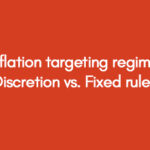
Inflation targeting regime: Discretion vs. Fixed rule.
February 27, 2021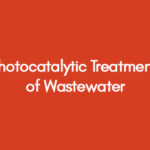
Photocatalytic Treatment of Wastewater
February 27, 2021Introduction
Construction of tunnels is considered to be one of the most important fields in civil engineering industry. The construction of tunnels is a highly technical work and it involves completion of various engineering tasks. Many important factors are considered to be involved in this field; therefore it is absolutely vital that the design and the consequent construction processes are completed in accordance with the international standards. Failure in any ‘one’ aspect of the overall system in place can cause serious damages.
In the initial stage of the tunnel construction, the proposed site is analysed and detailed reports are produced. During the site survey, the ground conditions are considered to be one of the most influential factors, as difficult ground conditions can slow down the advancing rate. Road tunnels provide a means of crossing physical barriers and water bodies. Tunnel construction mostly takes place when there is a need to pass a road network through industrial facilities, railroads, roadways and mountains. It could also be used to fulfil environmental requirements.
Figure 1: Glenwood Canyon Hanging Lake Tunnels
Construction of tunnels is also favourable when it comes to limiting air pollution, environmental damage and traffic congestion. Other good reasons for constructing tunnels include preservation of natural habitat, protection of private properties and historical sites and reduction in disturbance to surface land.
Road tunnels are considered to perform environmental friendly as compared other transport facilities, as these support in reducing traffic congestion. Thereby improving air quality because of traffic generated contaminants are captured and removed from the environment. Similarly, with use of tunnels the noise pollution will be lowered, and visual aesthetics and land use is improved. Property values and communities can be improved by moving to and underground traffic, thus resulting in less impact on the long term. Further, the tunnels provide the residents / users with the opportunity to develop land along the route and therefore the tunnel construction may provide boost to properties real estate influencing potential economic opportunities.
However, on many occasion, the wrong anticipation of weak rocks effects/behaviours on the tunnel construction process has caused problems in various tunnel construction projects. Accurate classification and determination of characteristics of such materials has been reported as difficult in various research articles and civil engineering reports. Therefore one of the objectives of this paper is to present a classification method when working with or expose with the weak rocks for the construction of tunnels
The purpose of this paper is to analyse the general tunnel construction methodology, best practices for tunnel construction and to provide the guild lines on weak rock classifications and characteristics.
Literature Review
The aim of this paper is to analyse the effective methods of tunnel construction and the effects of weak rocks. Much research has been completed in the last many decades to investigate and assess tunnel construction designs. This chapter aims to provide the tunnel design construction methods, planning and influential design parameters which must be considered for the successful construction of any tunnel.
Tunnel Design
Many researchers and engineers around the globe have emphasized on the need to adopt a reliable method of assessments and multi disciplinary involvement to come up with a good road tunnel construction design. Engineers are required to take into account several factors such as maintenance, stress requirements, size of vehicles, lighting provision, safety of motorists, construction cost, ventilation and operations. It should be noted that the life expectance of a tunnel should also be considered when determining the capital cost of construction and performing life cycle cost analysis.
Designing civil engineering tunnels in weak rocks has traditionally involved two separate activities. The first are those of a geotechnical engineers who carry out the site investigation, determine the rock mass characteristics and the load on the rocks, and calculate the stability of the tunnel. The second activity is that of the structural engineers, who carry out design of the final concrete lining on the basis of the loads provided by the geotechnical engineers. It is difficult to clearly distinguish between these two activities nowadays because there is need for structural design at various stages in the construction process and because the final lining design is directly impacted by this construction process. So the need to integrate the geotechnical and structural design components as part of a single activity is considered during many projects
Design Process
Design of a road tunnel uses the following principle processes:
- Defining the specification of requirements of the tunnel, including the layout of the proposal
- Defining the required life and durability of the tunnel.
- Carrying out critical analyses and investigative studies. The data includes results of the geotechnical, geologic and geohydrological analyses.
- Conducting the cultural, environmental, and institutional bases studies to analyze the impact of the tunnel on broader scale.
- Further comprehensive tunnel studies to figure out the most appropriate tunneling methodology
- Establishing the complete tunnel profile, the proposed cross section and alignment. This includes estimations and determination of critical aspects using various software and simulation tools and design calculations
- Completion of Engineering and Safety studies with respect to determination of potential failure modes which may also include unsatisfactory performance in the longer terms, construction events hazards and failure to comply with lows and regulation of environmental and safety standards. Necessary data must be collected from this study and these modes of failure must be analyzed in details.
- Completion of detailed risk analyses along with identification of mitigation measures, followed by their implementation with respect to practical and viable solutions.
- Preparing documents including plans for construction, schedules, and geotechnical baseline report (GBR), specifications, and various estimates.
Financial Studies
Depending on the life cycle cost analysis of a road tunnel, it is important to determine if it is financially viable. Tunnels are traditionally designed / constructed for a time span of around 90 to 130 years as it has been reported that throughout the world existing old tunnels which are more than hundred years old continue to operate successfully. There has been a recent trend in the tunnel construction industry to design the tunnels for a life of 150+ years. All costs must be in terms of life-cycle costs to help in comparison with a surface facility like a bridge. Net Present Value should be used to calculate the cost of construction, financing, operation and maintenance while calculating the life cycle cost of a tunnel construction project. Additionally, performing a cost-benefit analysis with respect to intangibles such as air quality, environmental benefits and impacts, noise pollution and vibration analyses, aesthetics, potential air tight and pressure developments, real estate, etc. can prove to be highly beneficial and influential.
Construction and operation risks / hazards should also be taken into account while performing the financial evaluation. Often, such risks and hazards are expressed as provisional cost items or financial contingencies. With advancement in the project design level, there will be a decrease in the level of contingencies. Henceforth, the risks are better quantified and the possible provisions to manage and control / reduce such risks and hazard can be identified.
Environmental and Community Issues
For the planning process, the impact of the construction on the community and the environment is important and should be addressed. Impact on normal traffic, community business, institutional blocks, residential areas, utilities, and healthcare facilities are some of the important issues which must be addressed. During the planning and design of the tunnel, the major impacts such as vibration during construction, construction noise, aesthetics, dust pollution, quality / supply of water and traffic congestion are major issues that need to be addressed and any possibility of adversely effecting the environment must be managed. An example of this can be noticed for false tunnel excavation requiring surface cover that has a direct impact on traffic, public services and facilities potentially close to site, which leaves a strip of land disturbed - the surface level, may require restoration and landscaping.
In urban cases, tunnels cut and cover can be problematic and can cut off access and public services in the short term. There will be need to provide alternative services for existing facilities during construction or , in the form other practical alternatives, which can route the construction process to allow access and maintain public. Construction of top-down rather than bottom-up construction can sometimes help relieve and reduce the scheduled disorders. Stiff excavation support systems and soil improvement techniques may be needed to minimize the potential settlements, lateral ground deformations, and its impact on adjacent structures. During the excavation process and removal of fluid/water near a contaminated soil, designated activities are necessary to avoid migration of the plume of groundwater in the excavation or its adjacent basements/surroundings.
Operational Issues
Provisions to address the operational and maintenance aspects of the tunnel and its facilities should be allowed when planning a tunnel. Issues such as control of traffic, lighting, life safety systems and emergency planning, ventilation system, tunnel cleaning, maintenance of equipment, and other such matters, should be identified during the initial planning phases. High maintenance equipment such as light fixtures can require frequent access and maintenance, and a good design would insure that such equipment can be accessed by the local control body with affecting the traffic in the tunnel. Similarly, equipments to carry out such activities much be provided to ensure that the overall project could be operated successfully.
Tunnels in Weak Rocks
Numerous challenges evolve while working with the weak rocks for the design and construction of tunnels. Weak rocks are known to poses low strength and high deformability characteristics and therefore can weak rocks are often over-stressed even when exposed to low level of stress. The characteristics of weak rocks can often lead to yielding, slapping/spalling, squeezing and ravelling conditions
Another important factor which must be taken into consideration is that stress value in the formations of weak rocks is not uniform or constant, and therefore there is always a possibility of deterioration when such formations are exposed in the tunnel designs. Furthermore, weak rocks are vulnerable to deformation with respect to age/time due swelling and squeezing conditions. All these unfavourable factors and conditions limit the advancing rates of tunnel construction and design and requires intense ground support.
Various classification procedures/systems have been developed in the recent past such as Q systems and Rock Mass Ratings; however they can only be applied rock masses which are jointed and for which the behaviour is analysed through discontinuities. Furthermore, these classifications do not specifically deal with the primary weak rock characteristics which are probability of overstress and deterioration. Therefore the classification method presented in the paper includes the two fundamental evaluation of characteristics which are the initial response of weak rocks and their behaviour in long term. It is worthwhile noticing that that important aspects and factors that must considered when working with rock includes evaluation of the rock type, mineral analyses, strength measurement, stress strain evaluation, stress level identification (in-situ), discontinuities and ground level water measurements.
The Uniaxial Comprehensive Measurement (UCS) method is used to analyse the weak rock characteristics of the intact weak rocks. According to the International Society of Rock Mechanics, also known as ISRM, have defined any rock as weak which has the UCS below 20 Mega Pascal (ISRM, 1981). Below this limit, upon shearing, the weak rock tend to contract instead of dilate. The materials which tend to dilate when sheared are generally considered to be stronger as they resist the imposed stress and therefore are less expected to deform and/or break.
The porosity, which is defined as the void space on surface of any material/rock, is another influencing factor with respect to the strength of the rocks. A material which has high porosity is generally believed to have low strength. Filling the void spaces of the material through cement and grains can reduce the porosity of the subject material, and hence increase the overall strength. According RETC Proceedings of 2001, mudstone with porosity of 10% or more, and sandstone of porosity greater than 20%, are like to be classified as weak rocks.
Some commonly known weak rock types include sedimentary rocks e.g. sand-stone, mud-stone, silt-stone etc, volcanic rocks e.g. breccia and tuff etc, and other altered/weathered rock types. It is important to note at this point that the weak rock conditions can also be produced through faulty rock mass, wrong jointing and shear zones.
Material Response
The imposed stress on the rocks in tunnel and the rock strength defines the initial response of any weak rock surrounded in the tunnel. The weak rock formation behaviour is dependent upon the weak strength and high capacity of deformability, however the underground water conditions and overall mass of rock can also have an influence on the behavioural response of weak rocks
Stress;
Tangential stress which is produced around the excavation of tunnel can test the streth of the surrounding weak rocks, which can also be another factor to the behaviour of weak rocks. If the induced stress of the tunnel excavation exceeds the maximum strength of the rocks, the tunnel rocks will fail or yield in response.
Groundwater;
Since the ground water has the ability to weaken the any rock, this phenomenon is more pronounced when working the weak rocks. The ground water can change the physical properties and the ground behaviour. Because of the discontinuities and the overstressing factor, the pressure of the ground water can rise around the tunnel excavation behind/adjacent to the blocks of rocks formations. This can results in serous damages and sometime complete failure of the tunnel construction as it may cause reduction in stability, lowering of the stand out time and complete fall out. The groundwater inflows can occur at locations where faulty zones are established, which can cause further instability of the rock mass and extension of voids on the exposed rocks. This problem can cause the selling and slaking of the rocks, which can adversely affect the long term performance of the exposed rocks
Tunnel Construction Methodology
Civil engineering team is required to perform various types of investigative activities in order to collect valuable geological, subsurface, structure and topographic data for successful planning, design and construction of a road tunnel. It should be noted that most geotechnical investigation procedures are same as those employed for bridges and road network construction. However, the focus of the investigation, aims, and the specific scope could be considerably different for tunnel and depend heavily on construction methods used and the subsurface conditions.
It is vital to use accurate investigation methods to be able to estimate the construction cost, time frame for project completion and construction risks. Furthermore, use of appropriate means and techniques can help in identification of properties and characteristics of the underground facilities. The time spent and money spent on geotechnical investigations are directly proportional to the project budget, objectives, scope and other constraints including environmental impact, geometry, third party limitations and constructability. In many cases, it is not possible to fully predict the ground behaviour or define exiting subsurface conditions and therefore all the involved parties should have a clear and common understanding of the risks associated to geotechnical investigations.
For best planning and design of a road tunnel project, the investigation programme should incorporate the following:
- Geologic Mapping
- Subsurface conditions
- Environmental impact
- Surveys and Site Reconnaissance
- Existing data collection and analysis
- Geospatial Data Management
- Seismicity
Tunnel Cross-Section
The configuration of tunnel cross-section's geometry/design must meet the mandatory traffic lane requirements, ensure adequate ventilation space, hard shoulders for safety of the vehicles, adequate lighting, ensure fire safety and traffic control systems. The tunnel construction method also dictates the cross-section. The use of tunnel boring machines for boring the tunnels will eventually produce circular configuration tunnel, whereas cut and cover construction methods will produce rectangular configuration, therefore it can be stated that the structural systems can be varied in accordance with requirements. Circular cross section has spaces available which can be used for home systems tunnel, as the vent or fan, traffic control systems and signals, lighting, closed circuit systems etc.
Several systems can be placed over the rectangular section, adjacent to traffic for cases when faced with the confined head space. In the intial stages of the tunnel design process, it is critically important that much consideration is given to the cross-sectional arrangement of the tunnel so to allow maintenance and inspection team an easy access throughout the tunnel so that the complete structure can be maintained in operational condition.
Invariably, the structural systems of any tunnel depend upon the selected method of construction and geometry of the cross section. This can be illustrated, for example, in the cut and cover tunneling, which produces a rectangular cross section, often the cast in-place concrete is the selected for the structural system, whereas SEM / NATM tunnel systems normally utilize shot-Crete and lattice-girders. Precast segmental single pass lining is a garrison used for soft ground tunnels. More often than not, the support system of the excavation can serve as the structural system of the tunnel end as the case in the construction of the top downwards method.
Cut & Cover Tunnels
In this section, we will be briefly discussing the challenges faced when using the cut and cover tunnel construction methodology. Cut and cover tunnel construction method involves use of backfill material to cover the excavation holding the tunnel structure. The backfill material is applied only when the structure construction is complete. It should be noted that this type of construction technique is suitable only when the excavation from the surface seems to be economically viable, acceptable and possible and the tunnel profile is shallow. Cut and cover tunnels are mostly constructed when there is a benefit in build tunnel at a shallow depth.
There are mainly two types of construction used when building a cut and cover tunnel including the top down method and the bottom up method. Again, the engineering team must prepare an effective plan and perform the required geotechnical investigations as part of the design process. The two constructions methods are briefly discussed under this section:
T
he use of cut and cover tunnel construction method is highly recommended when depth of 10 to 20 meters are desirable. Studies have revealed that this method turns out to be extremely favourable in terms and economy and practicality when dealing with depths mentioned above. The design of the cut and cover tunnel is usually based on a rigid frame box structure. If the neighbourhood is surrounded by urban areas, there may be a need to construct the tunnel using a tied back or braced excavation supporting walls and within a neat excavation line. Employing open cut construction has proved to be more economical when construction takes in open areas beyond urban development. When building tunnel over a city street, use of decking over the excavation is recommended to tackle the interference with traffic.
Figure 2: Cut and Cover Tunnel Bottom Up (a) and Top Down in (b)
Traditional Bottom Up Construction Method:
As shown in the Figure 3 below, the design of the conventional bottom up tunnel incorporates a trench which is excavated from the surface. Emphasising, the tunnel is built within the excavated trench, which is then covered with the back fill material. An excavation support system or an open cut is usually employed to form the trench.
Figure 3: Cut and Cover Tunnel Bottom Up (a) and Top Down in (b); Construction Sequence
Following are the steps of construction for the traditional bottom up method:
- Installing provisional excavation supporting walls such as lagging, tangent or secant pile walls, slurry walls, sheet piling and soldier pile.
- Trench dewatering as per the requirements.
- Use of struts or tie backs as provisional wall supporting components.
- Construction of floor to construct the tunnel.
- Building the walls and the roof of the structure and apply water proofing afterwards.
- Backfilling and restoration of the ground surface.
Following are advantages of the traditional bottom up method;
- Contractors better understand this method because it is the conventional construction method.
- There is easy access into the excavation for construction equipment and materials delivery, storage and installation.
- To channel water is deflected away from the structure and the drainage systems can be installed outside the structure.
- The outer surface of the structure can be waterproofed.
Following are disadvantages of the traditional bottom up method;
- Requires a larger base for the construction in comparison with the construction of the top down.
- It is not possible to restore the ground surface ti its final state until construction is complete.
- Requires utility relocation or temporary support.
- This method may require the removal of water. Surrounding infrastructure could be adversely affected by it.
Following are the steps of construction for the top down construction method:
- Installing excavation support for the tunnel’s structural walls.
- If required, dewatering within the excavation boundaries.
- Excavation requires till the bottom of the top slab of the tunnel.
- Construction and sealing the top slab of the tunnel; to the support of excavation walls.
- Restoring the surface of ground after completing the backfilling the roof.
- Excavating the tunnel interior. During excavation, the bracing of the excavation walls support is to be installed when required.
- Constructing the tunnel floor-slab.
- Finishing the primary and secondary walls
Advantages of the traditional top down construction method are:
- The excavation walls which served as the temporary support are also used as permanent walls of the structure.
- Ground surface can be restored earlier.
- The structural slabs used act as internal bracing for the support of excavation. Hence they reduce the quantity of required tie backs.
- Roof can be constructed with more ease since its casting can be done on prepared grade instead of having to use bottom forms.
- Less width is required for the construction area.
- Cost for the tunnel is lowered as a result of the elimination of the separate walls within the excavation.
- Construction activities can be can b completed side by side (overlapping), and it may shorten the construction duration.
- Cost decreases due to reduced need for internal bracing and tie backs.
Disadvantages of the traditional top down construction method are:
- Connections for the roof, floor and base slabs are much more complex.
- It is not possible to install external sealing / water proofing on the outer sides of the tunnel’s walls.
- There is a risk that the center columns or exterior walls will exceed the installation tolerances that are specified and therefore there would be a possibility of interior space extension
- There is a potential of water leaking at the joints, the ones between the walls and the slabs.
- Bottom slab excavation and construction has to be completed in limited space.
- Only limited access to excavation. Access only possible through designed shafts which connect to the roof
Groundwater Control
One of the main concerns of all the project management team is to construct a dry tunnel. A tunnel which is dry provides a safer and more environmental friendly conditions and considerably reduces maintenance and operating costs. In particular, advances in tunnel technology in recent years in the field of water-proofing have aided the implementation of strict criteria for water infiltration and the ability of building dry tunnels
Allowable Infiltration criteria followed by many countries such as UK and Australia is as below;
Tunnels ≤ 0.0020 gallons per square foot per day
Underground public space ≤ 0.0010 gallons per square foot per day
Moreover, no visible leakage or dripping from a single location shall be allowed. The water proofing systems in place for the tunnel designs are used to prevent entry of such water in underground opening consisting of a combination of various elements and materials. Such water proofing systems are designed through complete understanding and analyses of the geo-hydrological and geometrical conditions of the structure design and construction methods. Integrated sealing system should be used, taking into account the intermediate stages of construction, the final / latest structural conditions expected and the end use including operations and maintenance.
The most commonly us water proofing systems which are most commonly used are drained-open and undrained-closed. For these systems, various sealing materials are available. The open water proofing system inflow of water in a specific drain system
The area of the tunnel vault is provided with a sealing system which forms a protective umbrella drain water from seeping into the cavity. The drained-open systems are considered to be most useful where lower infiltration rates of water are expected.
The entrance of this water can occur at from various locations, such as fractures and joints, and the overall permeability is designed as such that the removal of the layers covering the soil underground rock mass is not affected in any way. Installation of this system is designed between the primary tunnel support side and the secondary / end support. Open water proofing mechanism normally allows enhanced economical coating and secondary reverse design, which considerably reduces the hydrostatic head.
Water proofing systems which designed as closed system can extend around the complete perimeter of the tunnel for the purpose of collecting the ground water flows into the proposed drainage system. Therefore the facility of ground water drainage is not provided in this system. Subsequently, the secondary coatings have to be considered for the building hydrostatic pressure of water. The application of this system is best suited on permeable soils where significant quantity ground water is discharged in the tunnel. Normally, segment linings are facilitated with a gasket to seal the joints between segments of prefabricated sections, therefore, providing a watertight tunnel. For tunnel section below the water table and submerged tunnels, waterproofing membranes are recommended
Fire Safety Systems -Life
Fire safety is considered to be extremely important in all the engineering works. Similarly for the construction of tunnels, it has be considered as the primary design parameter. Many incidents related to fire has been reported in the recent decades, in which the complete project has to face failure and it further caused fatal damages to the users of the tunnels. The disastrous consequences of fires in tunnels were reported in the tunnel of Mont Blanc in 1999 and the Swiss Gotthard in 2001, which unfortunately resulted in the loss human life and the property. It was reported that in fire incident at Gotthard tunnel, temperatures as high as thousand degrees centigrade was reached with a few minutes, and the fire spread of 2.5 kilometers (FHWA, 2006). This is due to the fact that heat in the tunnel is no dissipated and it spreads continuously until it reached the exit of tunnel. Incidents such as these highlighted the fact that the lack of fire safety in the construction process must not be compromised.
Therefore it vital that complete understanding of issues related to fire safety in the life of a road tunnel and consideration of their impact on the cross section of the tunnel, alignments, ventilation system in place, emergency exits and routes, estimated cost of the planning concept and the geometric configuration. Fire protection and safety requirements of the life of road tunnels by the National Fire Protection Association (NFPA) are as follows:
- Fire protection of the primary structural components and materials; must be considered at the initial stages of design. Multiple protective fire safety layers must be in place to ensure that the structural failure of the complete tunnel is avoided during an event of fire. Use of smart materials can also be utilized with state of the art technology when is much more stable at high temperatures
- Detection of fire methodology; must be comprehensively presented and installed at the designated locations. Fire detection systems such temperature measurement devices and smoke detectors are normally used for this purpose. These systems are electronically attached to the central control system. Upon receiving notification of high temperature or smoke, the activation of the following fire protection system is enabled automatically
- Fire protection equipment; such as water sprinklers, and other fire extinguishing systems must be installed. This is an extremely important activity as fire at the initial stages can be controlled with the use of such equipments. Fire hydrants, water supply lines and the fixed water emergency pipeline systems are utilized to extinguish the fire. These systems are provided with the high pressure water supply through the use of high pressure pumps at all times.
- Traffic Control; is another important factor which must considered for ensuring the safety of the tunnel. Uncontrolled traffic can cause serious accidents which can eventually cause breakage of serious fires and explosion. Such incidents can only be controlled through correct analyses of the expected traffic and subsequent control
- The systems of communication; has been reported to have failed on many incidents of fire. Communication systems are important as they allow timely notification of the fire and help in making emergency call to attain the outside help
- Emergency exits and access; provision of safe emergency exit is another important factor which has to be considered as the top priority when hazard analyses are conducted. The safe emergency exit allows a safe passage out of the fire emergency. It allows the fire safety teams an access to save lives and control the spreading of fire. These exits are carefully designed to ensure that enough space is allowed to ensure the safe passage to a full capacity of traffic under influence.
- Tunnel Drainage System; the drainage system may not seems to be as important as many other factors, however it is considered to be an important factor in the safe design parameters. The correct design drainage system must be able to ensure that flooding of the tunnel can be avoided during the case of emergency.
- Emergency response plan; emergency response planning is the plan of activities must be undertaken during emergency. The emergency response teams are required to plan their activities in such a way that corrective measures may be taken immediately upon notification of an emergency. Installation of base camps of teams such as ambulances and fire extinguishing teams can be a part of this planning.
Geometrical Configuration:
As highlighted before, the tunnel construction design depends on various factors such as the size of vehicles, stress requirements, ventilations, lighting and fire protection system. Therefore there is a need to consider additional geometrical requirements. It should be noted that factors such as design standards, horizontal and vertical alignments, travel clearance, cross section elements and traffic control requirements are required to be incorporated as part of the geometrical configuration.
Horizontal and Vertical Alignments
In this section, road tunnel horizontal and vertical alignment factors like horizontal and vertical curves, maximum grade, and other requirements and constraints are discussed.
Maximum Grades:
The grades of road tunnels must be evaluated with respect to the comfort of driver
While trying to maximize driver comfort, an economic balance point between operating and maintenance expenses and construction costs needs to be achieved.
In main roadway tunnels, it is preferable that the highest effective grades do not exceed 4 percent. For heavy vehicles, there may be a need for climbing lanes as a result of long or steep uphill grades. However, it is better to avoid climbing within tunnels, due to economic and ventilation reasons. Tunnel construction may also get considerably complicated by adding a climbing lane part-way through it.
Horizontal and Vertical Curves:
For a road tunnel, its horizontal alignment should be kept at minimum. Most of the tunnel length needs to be maintained on tangent as much as possible, as this will minimize the total required length, the operating efficiency will be improved, and the total numbers of required curves will be reduced / limited. Slight curves, however, may be needed in the design to ensure accommodation of specific ventilation systems and some other factors discussed earlier. To help drivers in a gradual adjust to the brightness outside the tunnel, a slight horizontal curve at the exit of the tunnel could prove to be beneficial.
The difference in elevation in the road surface between the inside to the outside edge of the roadway tunnel is called the super elevation rate. This should lie in the range one to six percent preferably. For walls with curved alignments when chorded construction is being employed, chord lengths should not be more than 25 feet for radii which are below 2,500 feet, and 50 feet elsewhere.
Distance Requirements for Sight and Braking
There are strict requirements for sight and braking distances in tunnels. It may be necessary to enlarge the tunnel locally to meet these requirements in the horizontal and vertical curves. This is done by providing a "sight shelf." Tunnels with extreme curvature have to be designed such that the sight distance must be examined carefully. Otherwise this may result in stopping sight distance being limited.
Other Considerations
Road tunnels over traffic tube should be designed so that in case a tube is closed, the traffic can be diverted to another facility. For safety reasons, it is suggested that the tunnels may be constructed for bidirectional traffic. It must be designed to be able to handle bidirectional traffic while maintenance work is being carried out, which must be able to perform in moments of high and low traffic volumes, such as at weekends and/or during office hours. Furthermore, it requires appropriate cutting areas which are usually located / provided outside tunnel’s entrance and exits, and the signaling and ventilation system must be designed to be able to cope with bidirectional traffic.
Geotechnical Investigations:
Working with weak rocks
Weak rock tunneling
Figure below shows a typical hard rock mass. The stability of relatively shallow civil engineering tunnels in such masses is controlled by rather simple structural features like joints, shear zones and faults. The intact rock pieces between these installed features in strong rocks simply act as rigid blocks. Thereby all of the movement and instability problems caused by this movement are controlled by the three-dimensional geometry which is associated with the intersection of these features and the excavation boundary. Preventing key blocks from being released and retaining the interlocking structure of the rock mass ensures the support of the excavations. To ensure that the excavations remain stable, we typically use combinations of rock bolts or cables and shotcrete linings.
Weak rock
Figures to be added
Rock masses in which their tectonic movement has resulted in the shearing and crushing of the original rock pieces or which may have disrupted any interlocking structure that may have existed are classified as weak rock masses. Figure below shows a typical example of this. A weak rock mass which has been further disrupted by natural or human processes like transportation, may become a soil or a waste rock pile. This, however, is an extreme case. These rock masses generally have a very low strength and hence failures can easily be induced in them by excavation of either tunnels or slopes. The characteristics of these rock masses, the strength and deformation characteristics estimates and the analysis of potential over-stressing problems in weak rock tunnels has been discussed by Hoek and Marinos (2000).
Figure below represents a summary of the many options that exist for tunnelling through weak rock masses. The method we chose depends on the size of the tunnel. all methods require both temporary lining for support during construction and final lining for the permanent operation of the project, apart from TBM tunnelling, which allows a pre-cast concrete final lining to be placed immediately behind the machine. One thing which is common to all of these methods is that at each excavation stage, it is necessary to create a completely closed structural shell, which is capable of carrying all the imposed loads.
Figures to be added
A detailed study of the various advantages and disadvantages of the different methods exceeds the scope of this paper. The following discussion involves a typical highway tunnel, the design of its temporary and final linings in three stages. In the first stage, we include the excavation of a top heading for the complete length of the tunnel. This is followed by removal of the bench in the second stage and finally the third stage of placing of the final concrete lining.
Figure: Conventionally driven tunnel shotcrete
Precast lining of concrete TBM driven tunnels
Further images/pictures to be added
Example
An example of a 12m span highway tunnel will be considered in order to illustrate the design of temporary and final linings. This example is similar o the many such tunnels that have been constructed in northern Greece, on the route of the 680 km long Egnatia highway. Altougha ny particular tunnel is not being considered here, these details are typical for tunnelling projects in the mountains along this route.
In figure 4, a simplified construction sequence is illustrated. It shows for the top heading, the excavation and its temporary support, the same for the bench and finally the placement of the permanent concrete lining. We must note that the concrete lining is being placed within a stable tunnel, and hence it is subjected to no loads other than its own weight. The final concrete lining is designed for the long term loading, which occurs as a result of the following:
- Loss of support due to corrosion. This load was provided by the rockbolts of other embedded steel elements.
- The properties of the temporary shotcrete lining getting deteriorated. the applicable design specifications, in many cases, require that when designing the final lining, any support provided by the rockbolts or temporary shotcrete lining have to be ignored.
- The surrounding rock mass properties getting deteriorated due to long-term chemical
alteration or creep.
- Water pressure around the tunnel building up due to long term blockage of the
drains. We should note that when the tunnel is located in a particularly sensitive
environmental region, long term drainage may be prohibited. Also, due to re-establishment of the groundwater table, the tunnel lining has to be designed for the full external water pressure.
- Live loads due to earthquakes, vehicle impact, fire, or explosions in the tunnel. Earthquake loads are generally ignored for tunnels with a vertical and lateral cover
of more than one diameter but we have to incorporate them into the design of very
shallow tunnels or for portals.
- Thermal stresses and shrinkage induced by the construction process and sequence.
In the design process we calculate the axial thrusts and the bending moments in both
the temporary and final linings at each construction stage and we have to check the maximum values against moment-axial thrust capacity diagrams to ensure appropriate reinforced shot creteor concrete diagrams. The design is an iterative process. We keep adjusting the amount of reinforcement and the thickness of the lining until the maximum moment-axial thrust combinations are acceptable. This is illustrated in the figures and text that follows.
Control of tunnel deformation
When we are driving a tunnel through a rock mass in an area where the ratio of rock mass strength to in situ stress is so low that failure develops around the tunnel (Hoek and Marinos, 2000), then deformation of the rock mass which occurs is similar to that shown in Figure 5. The first issue to be solved by the tunnel designer is the stability of the face of the tunnel. For large tunnels, we provide stablity by using grouted fibreglass dowels and/or forepole umbrellas.
Methodology
Softening/Slaking Potential
It has been seen that rocks which have high clay content are often prone to softening and slaking. This is caused by the moisture changes. Also, some sandstones are susceptible to slaking. Slaking results in the disintegration of the rock, whereas softening is a significant loss of strength. To ensure strength is maintained, rocks that will soften considerably when exposed to water must be protected. To determine the time-dependent loss of strength, strength tests are performed at various time intervals, and the potential for softening can be evaluated by soaking samples. It has been recorded that mudstones and shales have a UCS greater than about 3.4 MPa and they have been found to lose less than 40 percent of their original strength and they are not generally susceptible to significant softening (Morgenstern and Eigenbrod, 1974).
The mineralogic composition of the rock controls the potential for slaking, especially the presence of swelling clay minerals. Another important mechanism which produces cracks is stress relief in clay-bearing rocks. This allows water to penetrate therock mass more deeply.
Squeezing Ground
Squeezing is the collective name of time-dependent deformations. These deformations are associated with yielding, creep, or plastic behavior which is caused by overstressed
conditions in the rock mass around the tunnel. Squeezing has some effects which are
evident immediately after excavation, e.g. the convergence that occurs at thetunnel face. But there are also long-term effects of squeezing including continued ground movements and a gradual build up of load on the tunnel support system. The rock type, rock mass strength and deformation properties, and the in situ stress conditions determine the magnitude of the ground movements (i.e. tunnel convergence) associated with squeezing, ground loads, the extent of the yielding zone around the tunnel, and the support requirements. To address these issues, stress analyses and ground-lining interaction analyses are usually carried out. Approaches have been developed to do this and are discussed at other places (Deere et al., 1969; Jewtha et al., 1984). When characterizing the ground it is important to know the special requirements for these analyses. It is critical that the deformation properties of the rock mass be accurately determined in order to perform the analyses discussed above, . In situ tests we can use a dilatometer, a pressure meter, or Goodman jack can be performed to determine these properties. Additionally, triaxial tests may be mandatory to estimate strength parameters for these analyses.
Swelling Potential
Due to the adsorption of water there is an increase in volume of the rock mass surrounding the tunnel. This increased volume is known as swelling ground, and it displaces into the tunnel. Significant swelling pressures can develop when the volume increase is resisted. Swelling is mainly limited to the fine-grained rocks mentioned above. Swelling can also occur due to hydration of anhydrite, which produces gypsum or it may be due to the hydrothermal or chemical alteration of minerals such as feldspar, which produces montmorillinite Stress-induced cracking may help in access to water and thereby it can promote swelling. Swelling potential can be evaluated from the results of laboratory tests such as Atterberg limit determinations, free swell tests, X-ray diffraction evaluations which determine the type of clay minerals present and grain size analyses which determine the clay content (< 2 micron).
Results and Discussion
This section will be presented with the discussion on literature review and methodology. Detailed review of details such as rock tunnelling, mass characteristics summary of the weak and hard rocks and design parameters will be discussed. The tunnelling techniques and their successful implementation will also be included in this section. Further discussions and results analyses will be presented for highway tunnelling, tunnel deformation and control, weak rock determination methods, use of smart materials, lining capacity and lining designs.
Conclusion
Findings of the research conducted and the analyses completed will be presented in this section of the dissertation. A comprehensive summary of all the information, data, analyses and results will be concluded in this section.
References
Hoek, E. 2003. Integration of geotechnical and structural design of tunnel linings, pp 1-15. Available online at: [http://www.rocscience.com/assets/files/uploads/7694.pdf] (Accessed on 07/11/13).
Brady, B.H.G. and Brown, E.T. (1985). Rock mechanics for underground mining. London: Allen and Unwin.
Carranza, T. C. and Fairhurst, C. (1999). The Elasto Plastic response of underground excavations in rock masses that satisfy the Hoek-Brown failure criterion. Int. J. Rock Mech. Min. Sci. 36(6), 777–809.
Carranza, T. C. (2004). Elasto Plastic solution of tunnel problems using the generalized form of the Hoek-Brown failure criterion. In proc. ISRM
SINOROCK. (2004). Symposium China. Eds. J.A. Hudson and F. Xia-Ting). Int. J. Rock Mech. Min. Sci. 41(3), 480–481.
Carranza, T. C. (2004). Some Comments on the Application of the Hoek-Brown
MIR. (2004). Failure Criterion for Intact Rock and Rock Masses to the Solution of Tunnel and Slope Problems. Torino, (eds. G. Barla and M. Barla). Chapter 10, 285–326. Pàtron Editore.
Hoek, E. and Marinos, P. (2000). Predicting Tunnel Squeezing. Tunnels and Tunneling International. Part 1 and 2.
Hoek, E. (2001). Big tunnels in bad rock, Terzaghi lecture. ASCE Journal of Geotechnical and Geoenvironmental Engineering, Vol. 127, No. 9, pp.726-740.
Lunardi, P. (2000). “The design and construction of tunnels using the approach based on the analysis of controlled deformation in rocks and soils.” Tunnels and
TIPS. (2000).Tunneling International special supplement, ADECO-RS approach.
Sauer, G., Gall, V., Bauer, E and Dietmaier, P. (1994). Design of tunnel concrete linings using limit capacity curves. Computer Methods and Advances in Geomechanics, Eds.: Siriwardane & Zaman, page 2621 - 2626 Rotterdam, NL.
Wittke, W. (2000). Stability analysis for tunnels. Geotechnical Engineering in Research and Practice publication, WBI Print 4, Verlag Glückauf GmbH, Essen
Get 3+ Free Dissertation Topics within 24 hours?
Tunnels in Weak Rocks